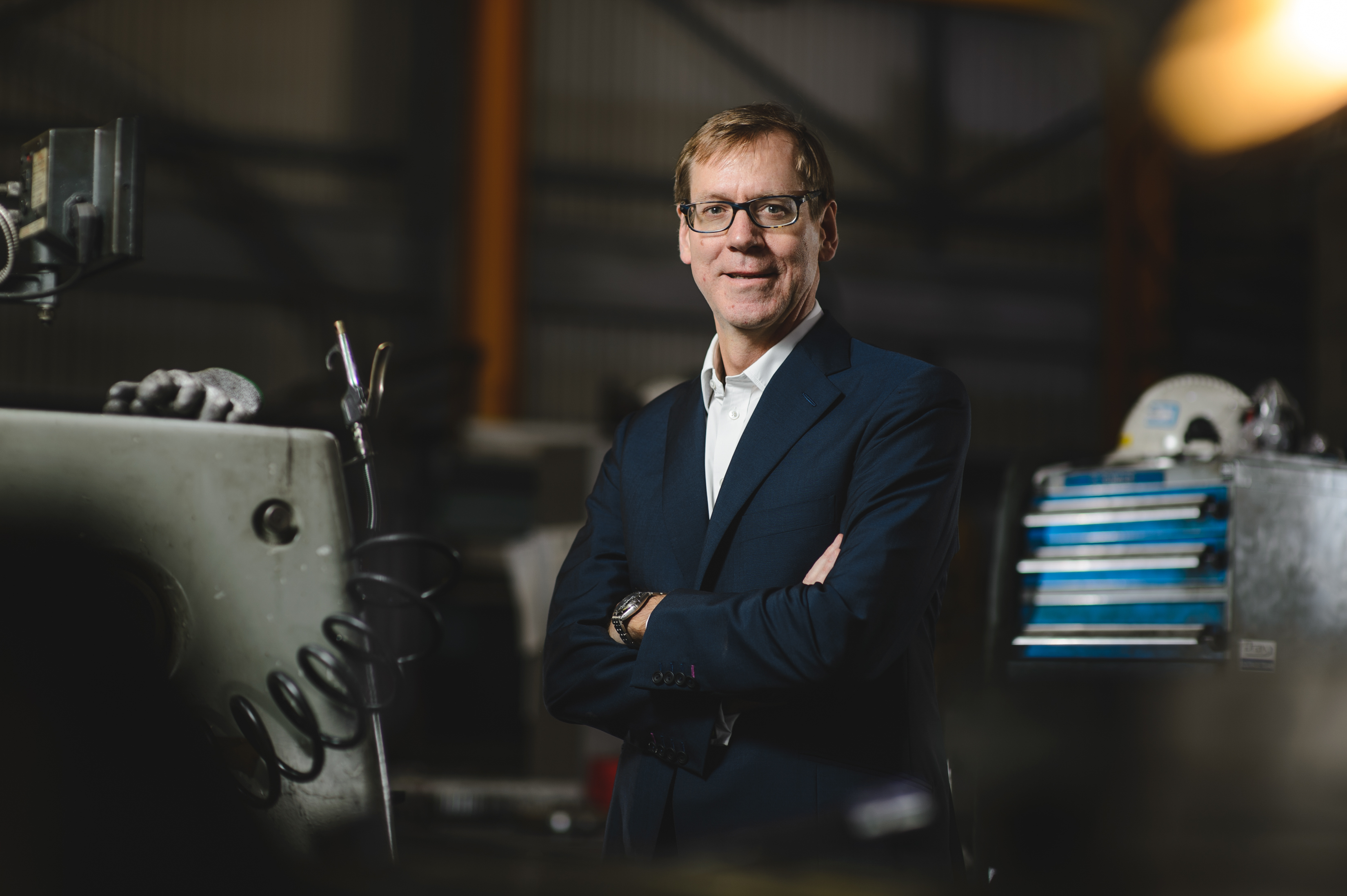
Climate change is the biggest challenge of our time and Drax has a crucial role in tackling it.
All countries around the world need to reduce carbon emissions while at the same time growing their economies. Creating enough clean, secure energy for industry, transport and people’s daily lives has never been more important.
Drax is at the heart of the UK energy system. Recently the UK government committed to delivering a net-zero carbon emissions by 2050 and Drax is equally committed to helping make that possible.
We’ve recently had some questions about what we’re doing and I’d like to set the record straight.
How is Drax helping the UK reach its climate goals?
At Drax we’re committed to a zero-carbon, lower-cost energy future.
And we’ve accelerated our efforts to help the UK get off coal by converting our power station to using sustainable biomass. And now we’re the largest decarbonisation project in Europe.
We’re exploring how Drax Power Station can become the anchor to enable revolutionary technologies to capture carbon in the North of England.
And we’re creating more energy stability, so that more wind and solar power can come onto the grid.
And finally, we’re helping our customers take control of their energy – so they can use it more efficiently and spend less.
Is Drax the largest carbon polluter in the UK?
No. Since 2012 we’ve reduced our CO2 emissions by 84%. In that time, we moved from being western Europe’s largest polluter to being the home of the largest decarbonisation project in Europe.
And we want to do more.
We’ve expanded our operations to include hydro power, storage and natural gas and we’ve continued to bring coal off the system.
By the mid 2020s, our ambition is to create a power station that both generates electricity and removes carbon from the atmosphere at the same time.
Does building gas power stations mean the UK will be tied into fossil fuels for decades to come?
Our energy system is changing rapidly as we move to use more wind and solar power.
At the same time, we need new technologies that can operate when the wind is not blowing and the sun is not shining.
A new, more efficient gas plant can fill that gap and help make it possible for the UK to come off coal before the government’s deadline of 2025.
Importantly, if we put new gas in place we need to make sure that there’s a route through for making that zero-carbon over time by being able to capture the CO2 or by converting those power plants into hydrogen.
Are forests destroyed when Drax uses biomass and is biomass power a major source of carbon emissions?
No.
Sustainable biomass from healthy managed forests is helping decarbonise the UK’s energy system as well as helping to promote healthy forest growth.
Biomass has been a critical element in the UK’s decarbonisation journey. Helping us get off coal much faster than anyone thought possible.
The biomass that we use comes from sustainably managed forests that supply industries like construction. We use residues, like sawdust and waste wood, that other parts of industry don’t use.
We support healthy forests and biodiversity. The biomass that we use is renewable because the forests are growing and continue to capture more carbon than we emit from the power station.
What’s exciting is that this technology enables us to do more. We are piloting carbon capture with bioenergy at the power station. Which could enable us to become the first carbon-negative power station in the world and also the anchor for new zero-carbon cluster across the Humber and the North.
How do you justify working at Drax?
I took this job because Drax has already done a tremendous amount to help fight climate change in the UK. But I also believe passionately that there is more that we can do.
I want to use all of our capabilities to continue fighting climate change.
I also want to make sure that we listen to what everyone else has to say to ensure that we continue to do the right thing.