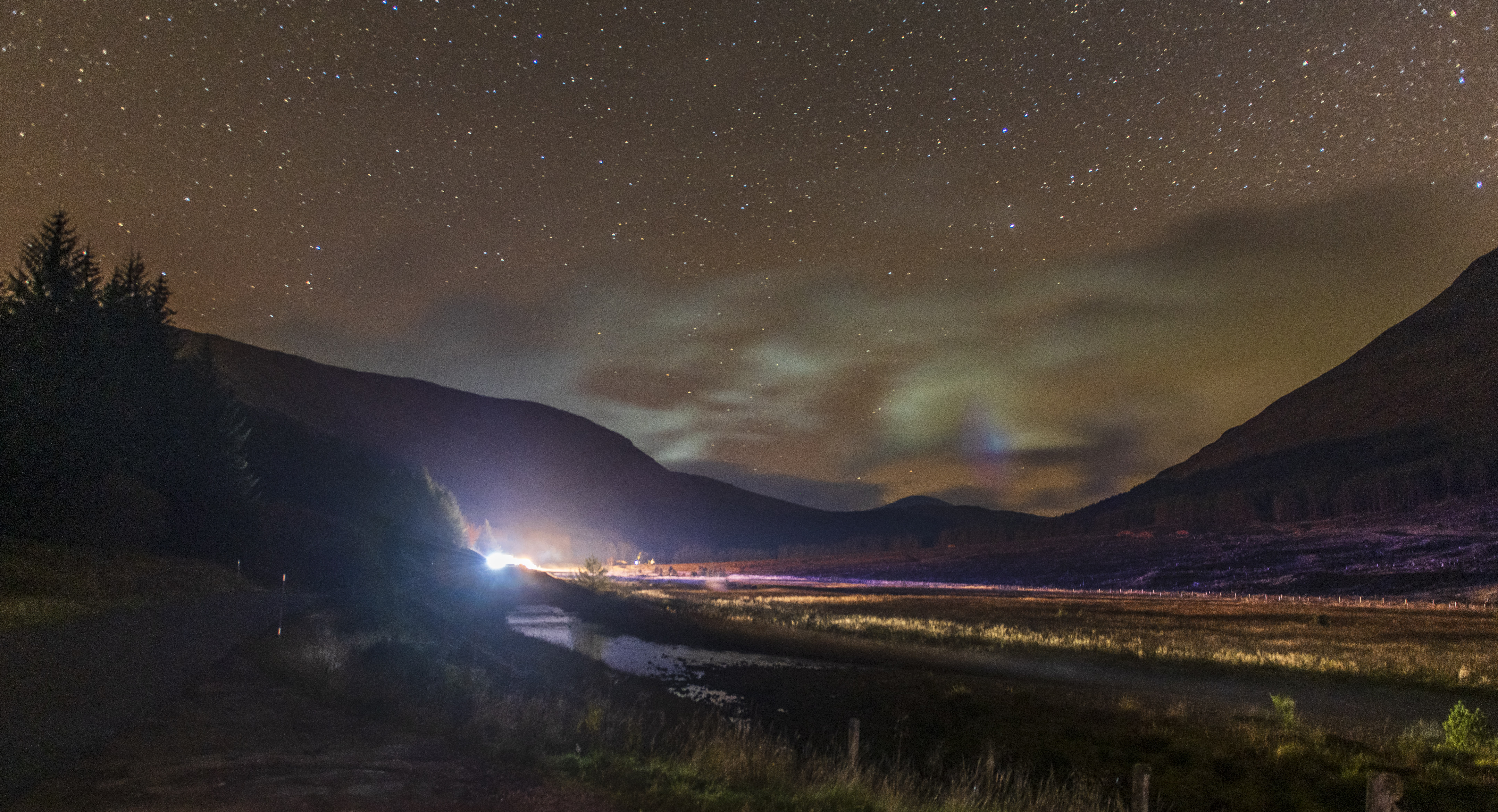
Nestled in in the Western Highlands in Scotland, Cruachan Power Station is surrounded by a breathtaking landscape of plunging mountainsides and curving lochs, between which weave narrow roads.
It makes for scenic driving. What might be trickier, however, is transporting 230 tonnes of electrical equipment up and down said mountains, navigating narrow bends.
But that’s exactly what a team from Drax was tasked with when it came to moving two 115 tonne transformers, the equipment used to boost electricity’s voltage. They were in storage 83 miles away at Longannet, currently being demolished, near Fife.
“You’re moving a piece of equipment that is designed to stay in one place. It’s not designed to go on the roads,” explains Jamie Beardsall, an Electrical Engineer from the EC&I Engineering team who worked on the project. “You’re very aware of your environment and the risks. Everything is checked and doubled checked.”
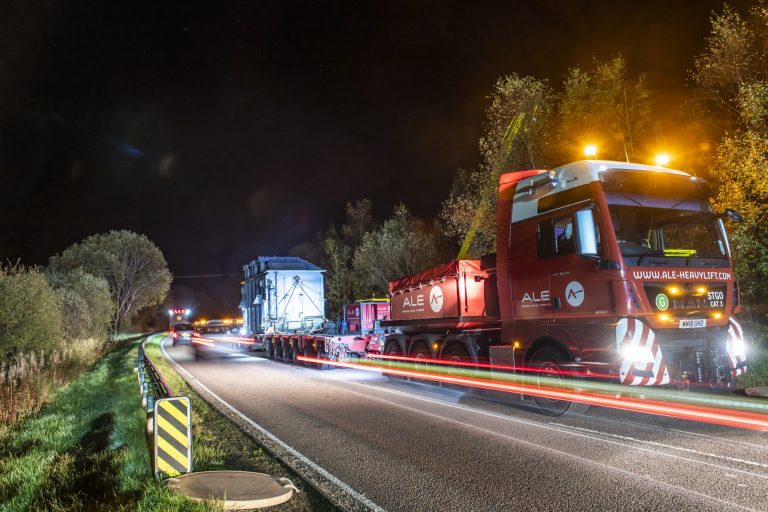
Transformers being driven to Drax’s Cruachan pumped storage hydro power station
The complicated task required colleagues from both Cruachan and Drax power stations to collaborate from the very beginning. Gary Brown, Mark Rowbottom and Jamie from the EC&I Engineering team based in Yorkshire teamed up with Gordon Pirie and Roddy Davies from Scotland who met frequently and planned the project alongside specialist transport contractor, ALE, which advised on heavy lifting and movement.
Planning and execution of the works also required constant liaison and coordination with the police and highway authorities in both Scotland and England. But more than that, the transformers’ one-by-one journey from the demolition site of what was once Europe’s biggest coal-fired power station, to a hydro-powered energy storage site on the other side of Scotland, represents the continual shift of Great Britain’s electricity away from fossil fuels.
Stepping up voltage
Transformers are an essential part of the electricity system. By increasing or decreasing the voltage of an electrical current they can enable it to traverse the national grid or make electricity safe to enter our homes.
“When we generate electricity, it is at a lower voltage than we need to send it out to the national grid,” says Beardsall. “We use transformers to increase the voltage so it can go out to the national grid and be transmitted over long distances more efficiently. We then reduce the voltage again so it can be brought safely into our homes.”
While all transformers apply the same principles for stepping voltage up and down, the two transformers that were transported through the Highlands to Cruachan were designed specifically for the pumped storage hydro power station, but stored at Longannet where there was more space. At the time, both stations where owned by Scottish Power. Cruachan was purchased by Drax on the last day of 2018.
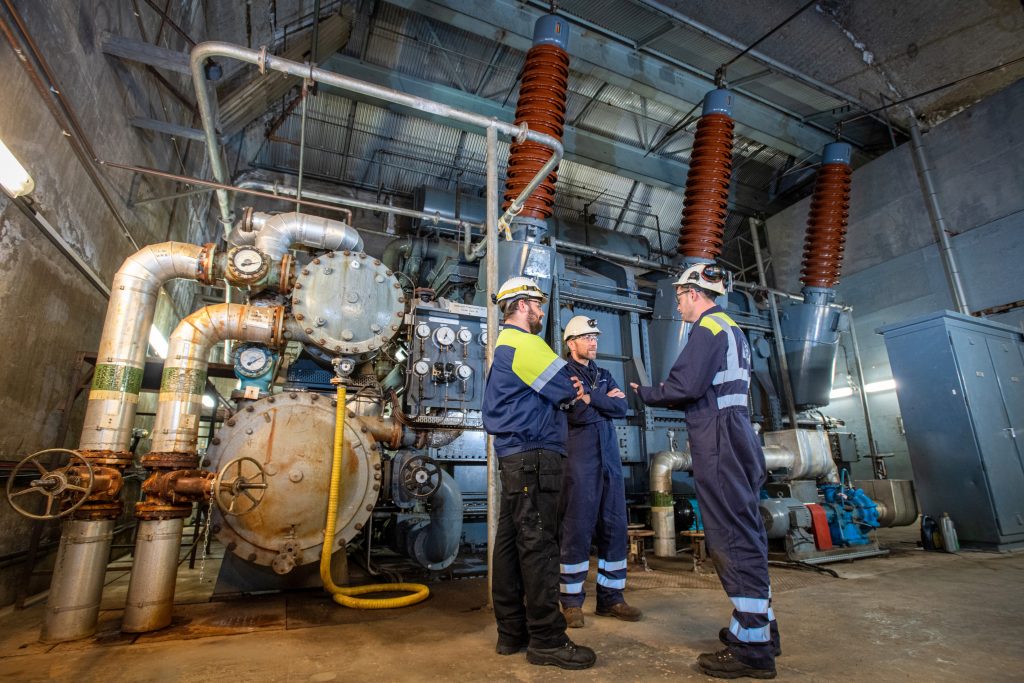
Engineers at Cruachan Power Station in front of one of the original transformers
When transported, each transformer weighs 115 tonnes and is almost four metres high. Transporting them isn’t as simple as loading them into the back of a van.
“You can’t transport them in a fully built state, they would be too heavy and wouldn’t go under bridges,” says Beardsall. “We had to strip them back to the core and now we’re working to reassemble them on site.”
Cutting down to the core
Each transformer consists of two main components; a core made of iron, and two windings made of copper. The transformer itself has no moving parts. When a voltage is applied to one of the transformer windings (the primary winding), a magnetic field is created in the iron core. This field then induces a voltage into the other winding (the secondary winding). Depending on the number of coils on each set of windings, the output voltage will increase or decrease. More coils on the secondary winding steps the voltage up, fewer coils on the secondary steps the voltage down.
This entire apparatus is submerged in an oil to provide insulation and keep the transformer cool, meaning the first step was to drain 50,000 litres of oil from each transformer. This was then sent to a refinery to be processed, cleaned and stored until the transformers are reassembled at Cruachan.
Oil removed, the Drax engineers oversaw and managed the dismantling of the transformers at Longannet. Once the transformers were stripped down to a state suitable for movement, they were loaded up one-by-one for transportation.
Meanwhile, at Cruachan, engineers worked on construction of a purpose built bunded area for storage of the transformers. The transformers were destined to be stored on land outside the main admin buildings, adjacent to Loch Awe.
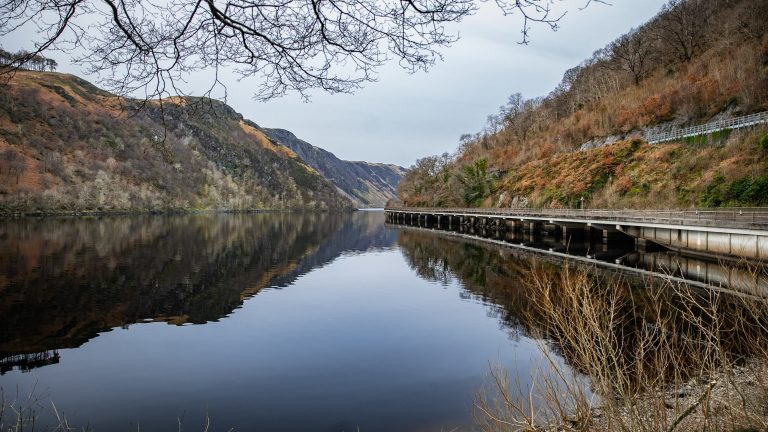
Loch Awe at Cruachan Power Station
The Loch itself is a beautiful place with abundant animal and birdlife – and a fish farm is located almost directly opposite the power station. In the event of a transformer leaking, the natural environment must be protected. An oil-tight storage area was therefore built, to ensure that no oil would end up in the Loch.
The road to Cruachan
Rather than heaving each of the transformers onto a trailer, each one was raised using hydraulic jacking equipment. A trailer was then driven underneath, and the transformer lowered onto it.
“The trailer is specifically designed to take the transformers and fit certain dimensions,” explains Beardsall. “It has 96 wheels over 12 sets of axles, each of which can be turned individually to assist in navigating around tight spots.”
The trailers are towed by large tractor units, each weighing over 40 tonnes. These provided the motive power to move the transformers. Each was moved in two stages over the space of two weeks. The first transformer over the course of a weekend, the second in the middle of the night some 10 days later.
“When we could go was governed by the police and highways agencies as they need to close the roads,” says Beardsall. “We set off from Longannet at 7pm on the Friday evening and moved them 60 miles along the route to a layby where we stored them. That leg took approximately five hours. Then the second leg was the last 25 miles to Cruachan, carried out on the Sunday morning of the same weekend.”
Navigating the Highlands with 115 tonnes of hugely valuable equipment is where the real challenge came in. Hills, dips and tight turns made for slow progress.
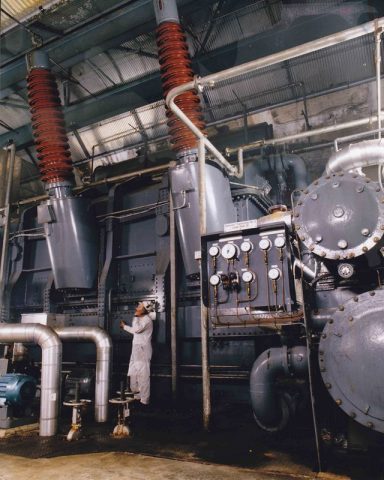
The original generator transformer at Cruachan Power Station
“The average speed was about 10mph, but we’re going through the Highlands so it was quite a bit slower than that in some places. We occasionally hit 20+ mph at points, but that was definitely for the minority of the time!” says Beardsall. “Some of the roads were so narrow it was difficult to get two cars past each other. The contractors also had to put metal plating over bridges because they weren’t strong enough to take the load.”
Having safely arrived at Cruachan, the transformers are being stored at surface level until they are needed, at which time they will be taken down the half-a-mile-long tunnel into the energy storage station.
“Typically a transformer has a design life of 25 years, although they can last longer” explains Beardsall. “There are four units at Cruachan and the transformers for two of these units have already been replaced, so these transformers would be used to replace the existing transformer for the two remaining units should it ever be needed. The existing transformer having been in operation since 1965.”
Moving heavy objects is part and parcel of running Drax’s multiple power stations around the country. However, navigating the Highlands, the very terrain which makes Cruachan possible, added a unique challenge for Drax’s engineers.