Tag: electricity generation
Maintaining electricity grid stability during rapid decarbonisation
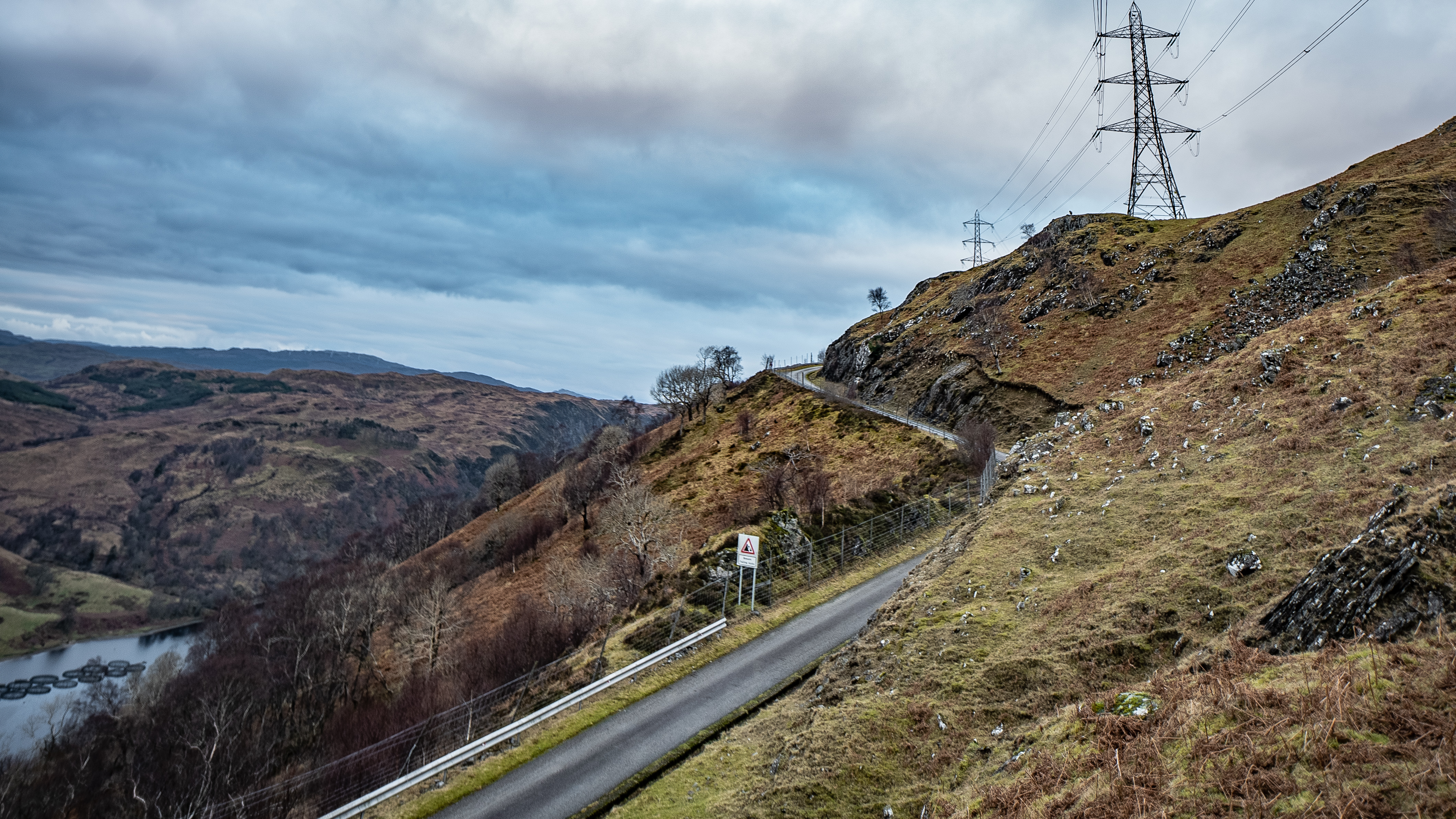
Great Britain’s electricity system is in the middle of a revolution. Where power supply was once dominated by some big thermal coal, gas and nuclear power stations, it now comes from an array of sources. Thousands of new individual points have been added to the mix, ranging from large interconnectors, that bring in power from neighbouring countries, through to wind farms, solar panels, small gas and diesel engines.
The energy mix has been changing radically, with low carbon sources expected to provide 58% of Great Britain’s power by 2020, up from 22% in 2010 and 53% in 2018. However, the security standards at which the electricity grid needs to be operated remain the same; these are predominantly voltage and frequency, and nominally 230 V and 50Hz for a domestic consumer.
The operation of the Transmission system, including maintaining these standards is overseen by the National Grid Electricity System Operator (ESO), using a set of vital tools it needs to have available, known as ancillary services. Some of this capability was inherent in large generators, which could provide the ancillary services required to keep a stable transmission system. Maintaining system stability, with thousands of generation points — a large part of which are not directly controllable — is increasingly challenging.
Ancillary services enable electricity to reach the end customer when and where it is required, in a safe manner, within acceptable quality standards. In addition to managing voltage and frequency levels, these standards also include maintaining adequate reserves to accommodate demand forecast uncertainties, generator breakdown and system faults. 1.
As the electricity mix changes, so must the process by which these services are secured. A diverse set of existing and genuinely new solutions will be needed to keep the lights on in the net zero carbon future.
Three steps to creating the right environment for a stable, resilient future grid:
1. Make the value of ancillary services transparent
In order for companies to help the ESO, be they generators or other service providers, it must be open and transparent about what’s needed to maintain grid stability and build resilience for the future.
“The ESO is the only buyer in the ancillary services market and is well-positioned to understand how the system is evolving. It should be proactively flagging how its needs may evolve in the future, so that the market can develop solutions to meet them”, says Marcelo Torres, Drax’s Regulation Manager – Markets.
Certain ancillary services still don’t have their own competitive markets and are provided as a “by-product” of the generation of electricity. An example is reactive power, for which there are no developed functioning regional markets yet. Generally, all power stations connected to the transmission network with a generation capacity of over 50MW are required to have the capability to provide this service, at a default price that may not reflect its real value to the system.
Another example is inertia, provided today largely through the heavy spinning turbines of thermal and pumped-storage hydro stations, which serve as stored energy that can slow down or smooth out sudden changes in network frequency.
If ancillary services were valued explicitly, market participants would have an insight into how much they are actually worth to the ESO and the grid’s stability, which would in turn incentivise new, competitive products to reach the market.
Torres points to technologies such as synchronous compensators, which are machines capable of providing ancillary services, including inertia and reactive power, without generating potentially unneeded electricity.
“These solutions will enable more renewables to connect safely to the network at a lower cost to consumers. For these solutions to come forward, ascribing the right value to ancillary services will be key. Without clear price signals, there is a risk of underinvestment in those technologies that provide the services needed, potentially resulting in price shocks for consumers”.
“The ESO is moving in the right direction with its recent Network Development Pathfinder projects. It has accelerated this work, launching its first ever tender for inertia and should roll out similar initiatives GB-wide. Such procurements should align with existing investment signals such as those provided by the Capacity Market. This should allow for the right type of capacity to be built where it is most needed, delivering a secure and resilient grid”.
2. Create market confidence
“Constructing the machinery and infrastructure that will enable the ESO to operate a carbon-free system will require major financial investment, as well as years to plan and build,” says Torres.
“This can only be achieved if Ofgem designs the ESO’s incentives in a way that rewards it for taking bold, strategic initiatives that have the potential to deliver good value for money to consumers in the long-term.”
Evidence of this working is shown in the success of offshore wind, which now provide around a sixth of Great Britain’s electricity, at record low prices. This is partly due to the government providing offshore wind developers with revenue stabilisation mechanisms, known as ‘Contracts for Difference’ (CfDs).
This is not a new concept for government and regulators around the world looking to enable investment in energy infrastructure. Financing renewables to achieve decarbonisation is enabled through CfDs or market-led hedging tools, like Power Purchase Agreements. Investment to ensure there is sufficient capacity to meet peak demand is secured through long-term contracts, competitively awarded through the Capacity Market. Similarly, investment in interconnection is supported through Ofgem’s ‘Cap & Floor’ regime.
“Subsidy isn’t required for investment in ancillary services. What’s needed,” says Torres, “is a clear and stable market framework designed around the system’s needs, which provides a mix of short and long-term signals. More certainty over the market landscape and the expected returns will lower the risk of these investments and get the solutions needed at a lower overall cost to consumers.”
“Long-term procurement is not the right answer everywhere. Where there is already a mature and liquid market, such as the case for frequency response, buying services closer to real time makes sense for two reasons. First, it allows prices to reflect more accurately the market conditions and therefore the real value of a service at the time when it is needed. Second, it allows a wider range of resources to participate in the market, increasing competition. Striking the right balance between short and long-term procurement is key to create a sustainable ancillary services market.”
Currently, the ESO requests that electricity-generation firms commit to supplying a certain amount of power for the purpose of frequency response, a month ahead of time. For resources such as wind farms or solar, which are dependent on the weather, this makes it extremely difficult for them to enter this market. Even for conventional large thermal generators it can be a problem, as many of them do not know how or if they will be running beyond a few days.
“The ESO is currently conducting some trials procuring frequency response one week in advance. While this is an improvement, it is still too long a lead time for intermittent sources or demand-side response, which ideally need day-ahead or almost real time auctions to unlock their full potential,” says Torres.
“The ancillary services market has been through a prolonged period of change since the ESO published its System Needs and Product Strategy in 2017. Without knowing how the market landscape will look like by the end of these reforms, it’s difficult for providers to develop the right solutions.”
A shift in thinking, which considers what the electricity network might require in the future, and how to provide the market with financial incentives to make it a reality, is needed. A resilient, stable future system is to the advantage of consumers.
3. Diversify
There will be no silver bullet that can solve all the challenges the energy transition poses. Maintaining system reliability in a high renewables world will require large amounts of dispatchable power, with different response time and duration. From small batteries and demand-side response that will manage instantaneously frequency fluctuations, through to large pumped storage hydro plants that will provide backup power during the days when the wind won’t blow and the sun won’t shine. A framework structured around the system’s different needs should aim at harnessing flexibility across a range of technologies and sizes.
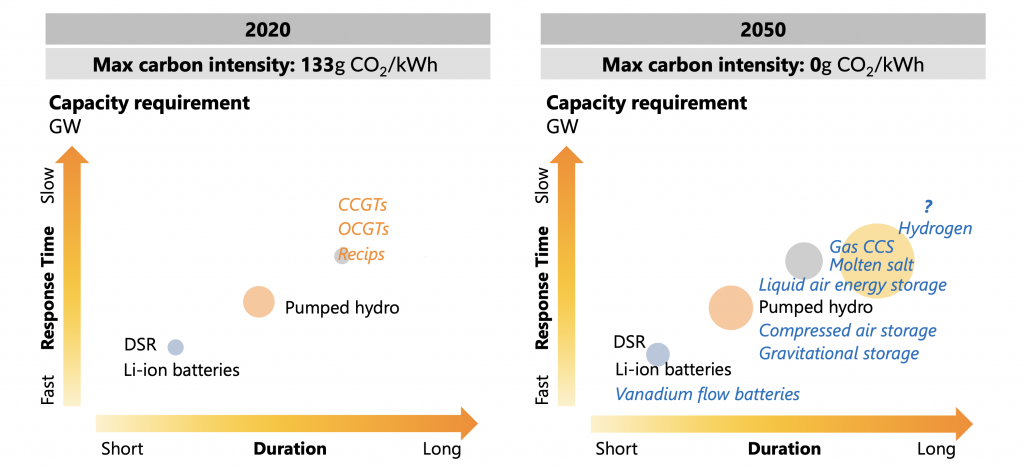
Source: The road to 2050: The need for flexibility in a high renewables world, by Ana Barillas, Aurora (2019)
Truly diversifying will also involve unlocking the flexibility potential on the distribution grid. To achieve this, the way that access to the distribution network is managed and paid for will need to evolve. Today, with big parts of the distribution network being congested, small flexible assets are asked to wait in the queue for several years or face disproportionate amount of network reinforcement costs to get connected.
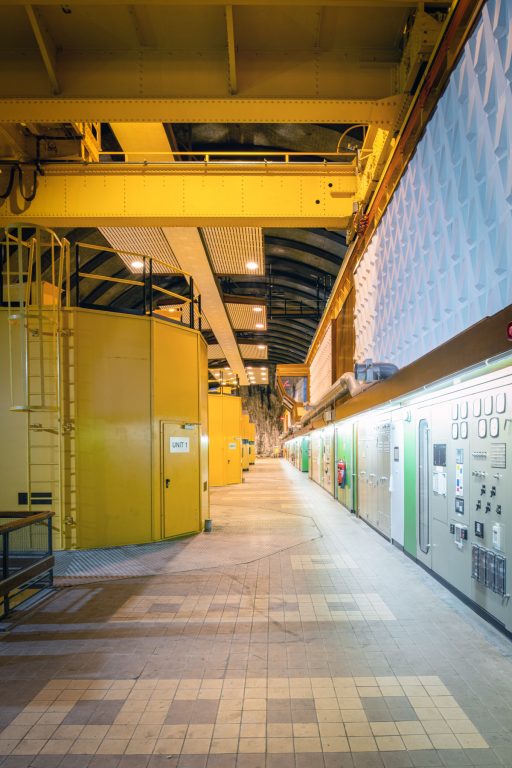
Machine hall, Cruachan Power Station
The ongoing review of the network access and forward-looking charging arrangements needs to address these barriers soon, if we are serious about making use of flexibility to foster the energy transition, while keeping consumer bills as low as possible.
Since 2018, GB’s Distribution Network Operators (DNOs) have been tendering and procuring for various flexibility services to manage congestion in regional electricity grids. In 2019, they published a roadmap setting out the steps they intend to take to enable a smarter and more flexible energy system.
“As we transition from DNOs towards Distribution System Operation – a wider set of functions and services to run a smart distribution grid – the regional networks will be open to market-based flexibility solutions. DSOs will be able to compete on a level playing field, offering options for network reinforcement. As DNOs move from trials to more structured flexibility procurement, harmonisation and effective coordination with the national markets will be the key pre-requisites to reveal the true value that flexibility can bring to the energy system,” argues Torres.
“To build a modern and resilient grid we will need a wider lens on what’s possible. It’s going to be an exciting journey on the road to net zero!”
This story is part of a series on the lesser-known electricity markets within the areas of balancing services, system support services and ancillary services. Read more about black start, system inertia, frequency response, reactive power, voltage control and reserve power. View a summary at The great balancing act: what it takes to keep the power grid stable and find out what lies ahead by reading Balancing for the renewable future.
What is a fuel cell and how will they help power the future?
How do you get a drink in space? That was one of the challenges for NASA in the 1960s and 70s when its Gemini and Apollo programmes were first preparing to take humans into space.
The answer, it turned out, surprisingly lay in the electricity source of the capsules’ control modules. Primitive by today’s standard, these panels were powered by what are known as fuel cells, which combined hydrogen and oxygen to generate electricity. The by-product of this reaction is heat but also water – pure enough for astronauts to drink.
Fuel cells offered NASA a much better option than the clunky batteries and inefficient solar arrays of the 1960s, and today they still remain on the forefront of energy technology, presenting the opportunity to clean up roads, power buildings and even help to reduce and carbon dioxide (CO2) emissions from power stations.
Power through reaction
At its most basic, a fuel cell is a device that uses a fuel source to generate electricity through a series of chemical reactions.
All fuel cells consist of three segments, two catalytic electrodes – a negatively charged anode on one side and a positively charged cathode on the other, and an electrolyte separating them. In a simple fuel cell, hydrogen, the most abundant element in the universe, is pumped to one electrode and oxygen to the other. Two different reactions then occur at the interfaces between the segments which generates electricity and water.
What allows this reaction to generate electricity is the electrolyte, which selectively transports charged particles from one electrode to the other. These charged molecules link the two reactions at the cathode and anode together and allow the overall reaction to occur. When the chemicals fed into the cell react at the electrodes, it creates an electrical current that can be harnessed as a power source.
Many different kinds of chemicals can be used in a fuel cell, such as natural gas or propane instead of hydrogen. A fuel cell is usually named based on the electrolyte used. Different electrolytes selectively transport different molecules across. The catalysts at either side are specialised to ensure that the correct reactions can occur at a fast enough rate.
For the Apollo missions, for example, NASA used alkaline fuel cells with potassium hydroxide electrolytes, but other types such as phosphoric acids, molten carbonates, or even solid ceramic electrolytes also exist.
The by-products to come out of a fuel cell all depend on what goes into it, however, their ability to generate electricity while creating few emissions, means they could have a key role to play in decarbonisation.
Fuel cells as a battery alternative
Fuel cells, like batteries, can store potential energy (in the form of chemicals), and then quickly produce an electrical current when needed. Their key difference, however, is that while batteries will eventually run out of power and need to be recharged, fuel cells will continue to function and produce electricity so long as there is fuel being fed in.
One of the most promising uses for fuel cells as an alternative to batteries is in electric vehicles.
Rachel Grima, a Research and Innovation Engineer at Drax, explains:
“Because it’s so light, hydrogen has a lot of potential when it comes to larger vehicles, like trucks and boats. Whereas battery-powered trucks are more difficult to design because they’re so heavy.”
These vehicles can pull in oxygen from the surrounding air to react with the stored hydrogen, producing only heat and water vapour as waste products. Which – coupled with an expanding network of hydrogen fuelling stations around the UK, Europe and US – makes them a transport fuel with a potentially big future.
Fuel cells, in conjunction with electrolysers, can also operate as large-scale storage option. Electrolysers operate in reverse to fuel cells, using excess electricity from the grid to produce hydrogen from water and storing it until it’s needed. When there is demand for electricity, the hydrogen is released and electricity generation begins in the fuel cell.
A project on the islands of Orkney is using the excess electricity generated by local, community-owned wind turbines to power a electrolyser and store hydrogen, that can be transported to fuel cells around the archipelago.
Fuel cells’ ability to take chemicals and generate electricity is also leading to experiments at Drax for one of the most important areas in energy today: carbon capture.
Turning CO2 to power
Drax is already piloting bioenergy carbon capture and storage technologies, but fuel cells offer the unique ability to capture and use carbon while also adding another form of electricity generation to Drax Power Station.
“We’re looking at using a molten carbonate fuel cell that operates on natural gas, oxygen and CO2,” says Grima. “It’s basic chemistry that we can exploit to do carbon capture.”
The molten carbonate, a 600 degrees Celsius liquid made up of either lithium potassium or lithiumsodium carbonate sits in a ceramic matrix and functions as the electrolyte in the fuel cell. Natural gas and steam enter on one side and pass through a reformer that converts them into hydrogen and CO2.
On the other side, flue gas – the emissions (including biogenic CO2) which normally enter the atmosphere from Drax’s biomass units – is captured and fed into the cell alongside air from the atmosphere. The CO2and oxygen (O2) pass over the electrode where they form carbonate (CO32-) which is transported across the electrolyte to then react with the hydrogen (H2), creating an electrical charge.
“It’s like combining an open cycle gas turbine (OCGT) with carbon capture,” says Grima. “It has the electrical efficiency of an OCGT. But the difference is it captures CO2 from our biomass units as well as its own CO2.”
Along with capturing and using CO2, the fuel cell also reduces nitrogen oxides (NOx) emissions from the flue gas, some of which are destroyed when the O2and CO2 react at the electrode.
From the side of the cell where flue gas enters a CO2-depleted gas is released. On the other side of the cell the by-products are water and CO2.
During a government-supported front end engineering and design (FEED) study starting this spring, this CO2 will also be captured, then fed through a pipeline running from Drax Power Station into the greenhouse of a nearby salad grower. Here it will act to accelerate the growth of tomatoes.
The partnership between Drax, FuelCell Energy, P3P Partners and the Department of Business, Energy and Industrial Strategy could provide an additional opportunity for the UK’s biggest renewable power generator to deploy bioenergy carbon capture usage and storage (BECCUS) at scale in the mid 2020s.
From powering space ships in the 70s to offering greenhouse-gas free transport, fuel cells continue to advance. As low-carbon electricity sources become more important they’re set to play a bigger role yet.
Learn more about carbon capture, usage and storage in our series:
- Planting, sinking, extracting – some of the ways to absorb carbon from the atmosphere
- From capture methods to storage and use across three continents, these companies are showing promising results for CCUS
- Why experts think bioenergy with carbon capture and storage will be an essential part of the energy system
- The science of safely and permanently putting carbon in the ground
- The numbers must add up to enable negative emissions in a zero carbon future, says Drax Group CEO Will Gardiner.
- The power industry is leading the charge in carbon capture and storage but where else could the technology make a difference to global emissions?
- A roadmap for the world’s first zero carbon industrial cluster: protecting and creating jobs, fighting climate change, competing on the world stage
- Can we tackle two global challenges with one solution: turning captured carbon into fish food?
- How algae, paper and cement could all have a role in a future of negative emissions
- How the UK can achieve net zero
- Transforming emissions from pollutants to products
- Drax CEO addresses Powering Past Coal Alliance event in Madrid, unveiling our ambition to play a major role in fighting the climate crisis by becoming the world’s first carbon negative company
How the market decides where Great Britain gets its electricity from
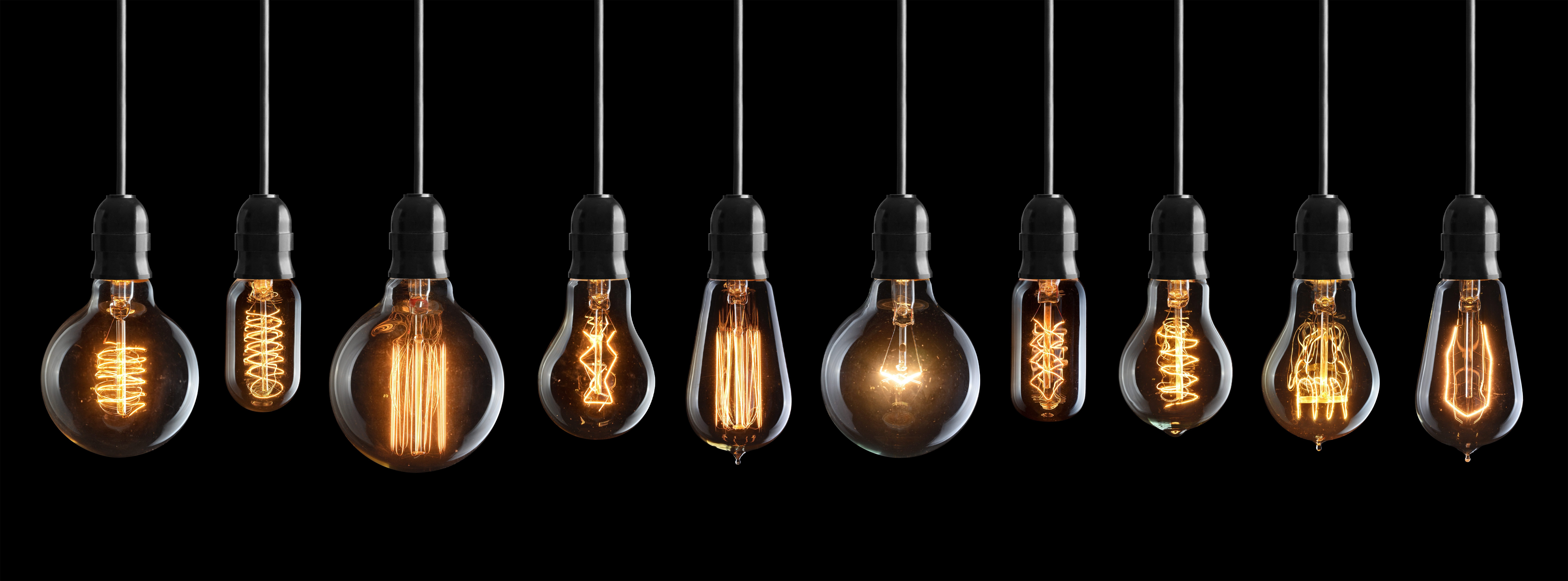
The make-up of Great Britain’s power system changes constantly. Demand is always changing; in winter it may peak at 50 gigawatts (GW) but overnight in summer it will be less than 20 GW. Some days wind is the biggest source of the country’s electricity generation, other days it’s gas. Then there are days when, for a few hours, solar takes the top spot in the middle of the day and nuclear during the night.
In the past, Great Britain’s electricity came almost entirely from big coal and nuclear power stations. But as the need for decarbonisation has grown, so has the number of sources feeding electricity to the grid, creating an ever more complex and varied system made up of technologies that behave in very different ways. For example, some sources are weather dependent and can’t generate all day every day others stop and start flexibly to smooth out changes in demand or intermittent generation.
But in the event all sources are available, what dictates which sources actually generate, and when? The overriding influence is economics – the costs of starting up and running a turbine, the price of fuel or taxes on carbon emissions.
In Great Britain, electricity’s wholesale price is not set in stone by an entity such as a regulator. Instead it’s negotiated via trading over the course of a day between generators (power stations, storage and wind turbines) and suppliers, who transmit that electricity to consumers.
As a result, the price of electricity fluctuates every half hour, responding to factors such as demand, cost of fuels, availability of resources (such as sun and wind), and carbon taxes.
For an example of the scale at which it fluctuates, we can look at the period 1-4 June this year, when the index price of electricity ranged from over £55/MWh down to around £5/MWh (see chart, above).
But while these figures speak to the overall price of a megawatt on the system, they don’t reflect all the individual sources, nor their individual costs. Each of the multiple sources on the grid have their own operating costs fluctuating on a similar basis.
These changing prices give rise to what is known as the merit order, a fluid, theoretical ranking of generation sources. This is not set by any regulator, economist or even by traders. Rather it is a naturally occurring, financial occurrence that explains what sources of electricity generation are feeding power onto the grid day-to-day.
What is the merit order?
The merit order dictates which sources of generation will deliver power to the grid by ranking them in ascending order of price together with the amount of electricity generated. This then determines the order in which power sources are brought onto the system. Ultimately, suppliers want the right amount of electricity for the best possible price, so in a system made up of many sources, it is the lowest cost, highest yield option that is brought online first, which in theory helps keep overall electricity prices down.
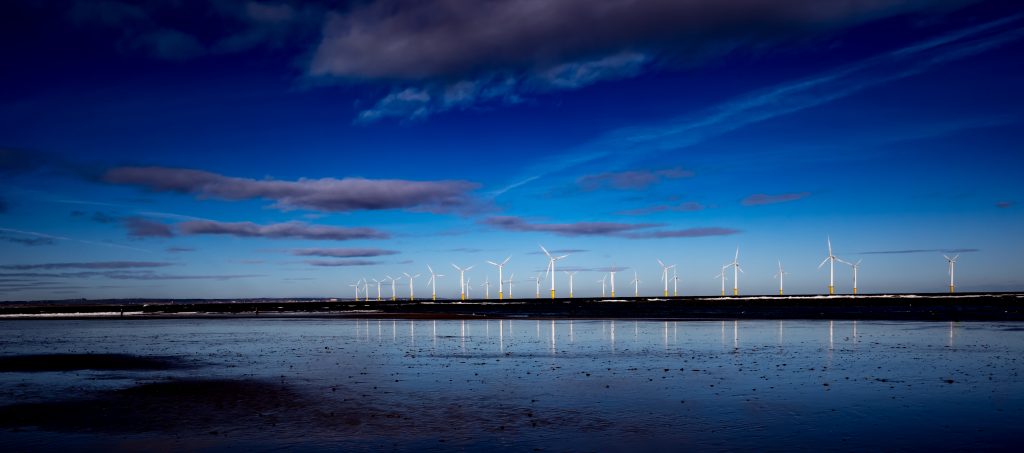
North Sea Wind Farm, Redcar
This means it is often sources such as wind and solar, which have no fuel costs, that sit at the top of the merit order. Nuclear may come next as it continually generates a large amount of power for a low cost, while taking a long time to turn down or off. At the opposite end of the merit order are sources like coal and oil, which have high fuel and carbon dioxide (CO2) emission costs (such as carbon taxes and the European Emission Trading scheme).
However, the merit order isn’t a set of hard and fast rules. “It’s an assumption used by traders or market commentators to guide what is likely to run and thereby the likely market price,” says Ian Foy, Drax Head of Ancillary Services. “There is no published merit order. It is like Santa Claus – it doesn’t exist, but it makes explaining Christmas easier.”
The intricacies of being in and out of merit
If a generating unit is required to meet demand then it’s described as ‘in merit’, if it is not required at any particular point in time then it’s ‘out of merit’ – there’s no point in suppliers paying for another power station, for example, to start generating if demand is already being met.
“If the market is efficient, we generate from the lowest cost source at all times,” says Foy. “Costs are not simple, for example, you have to take into account the cost of starting or shutting down generating units. However, costs are not publicly shared so there’s no single view of the merit order. Each party has its own perspective on it.”
It means the merit order changes from season-to-season, day-to-day and hour-to-hour, as rates of supply and demand, and the availability of resources change.
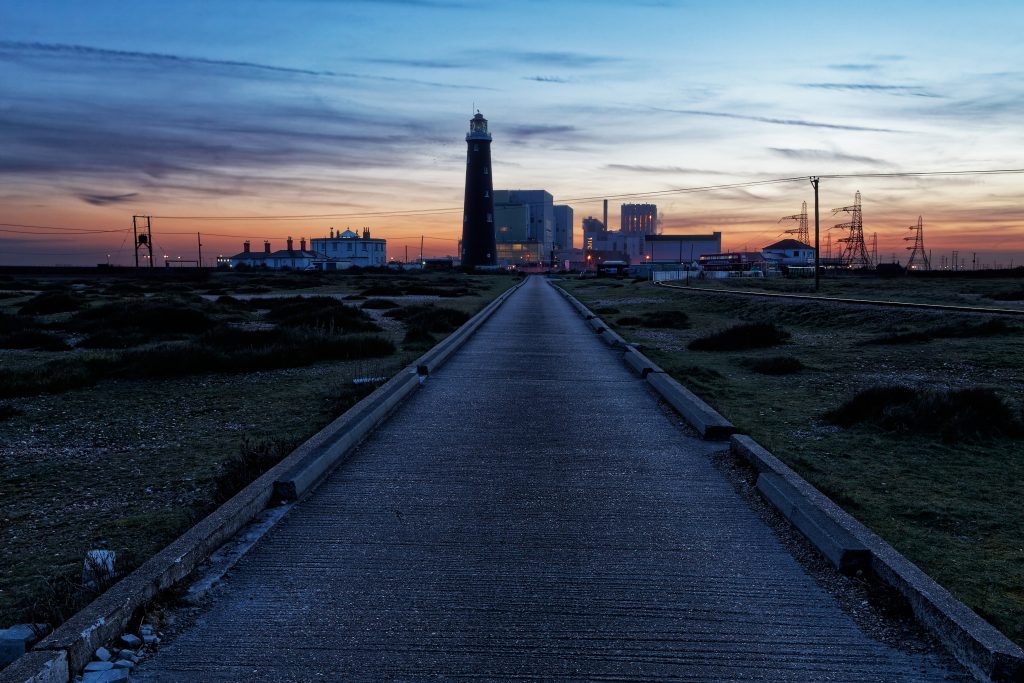
Dungeness Nuclear Power Station in Kent
“An obvious example is gas tends to be cheaper in summer than winter, when it’s not being used for heating. Coal and gas also switch as global prices change,” says Foy. “Availability also changes over the year. There’s more solar in summer, but none in the morning and evening peaks of winter.”
There are also practical issues, such as repairs being made on wind and hydro turbines or planned maintenance outages on thermal and nuclear power stations, putting those generators out of action and knocking them out of merit.
And because the merit order is not an implemented working scheme, it can be deliberately manipulated by outside forces. One of the ways this is most clearly seen is in carbon pricing.
Merit in a changing system
The Carbon Price Support tax paid by coal and gas generators in Great Britain, alongside the European Emissions Trading System have increased the cost of fossil fuel generation: gas, oil and coal. Levied as £/tonne of CO2 emitted this has the effect of pushing fossil generation down the merit order. With coal emitting double the CO2 per unit of electricity compared to gas, we can see how the merit order can be influenced to achieve environmental outcomes.
This has helped steer Great Britain towards record breaking coal-free periods and stimulated the building of low carbon generation sources.
Other sources, such as interconnection with Europe and power storage facilities, also slot into the merit order. Their position often shifts due to highly variable prices dependent on power generation in neighbouring countries or the amount of electricity that can be stored at a low cost, respectively.
The grid is ever-changing. Over the last two decades we’ve seen huge shifts in how power is generated and delivered. This is unlikely to slow down in the near future, but the merit order will remain. Like the grid, it is in a constant state of change, adapting to the many moving parts of the electricity system. As long as Great Britain maintains its open electricity trading market, the merit order will continue to dictate where the country’s power comes from.
What makes a mountain right for energy storage
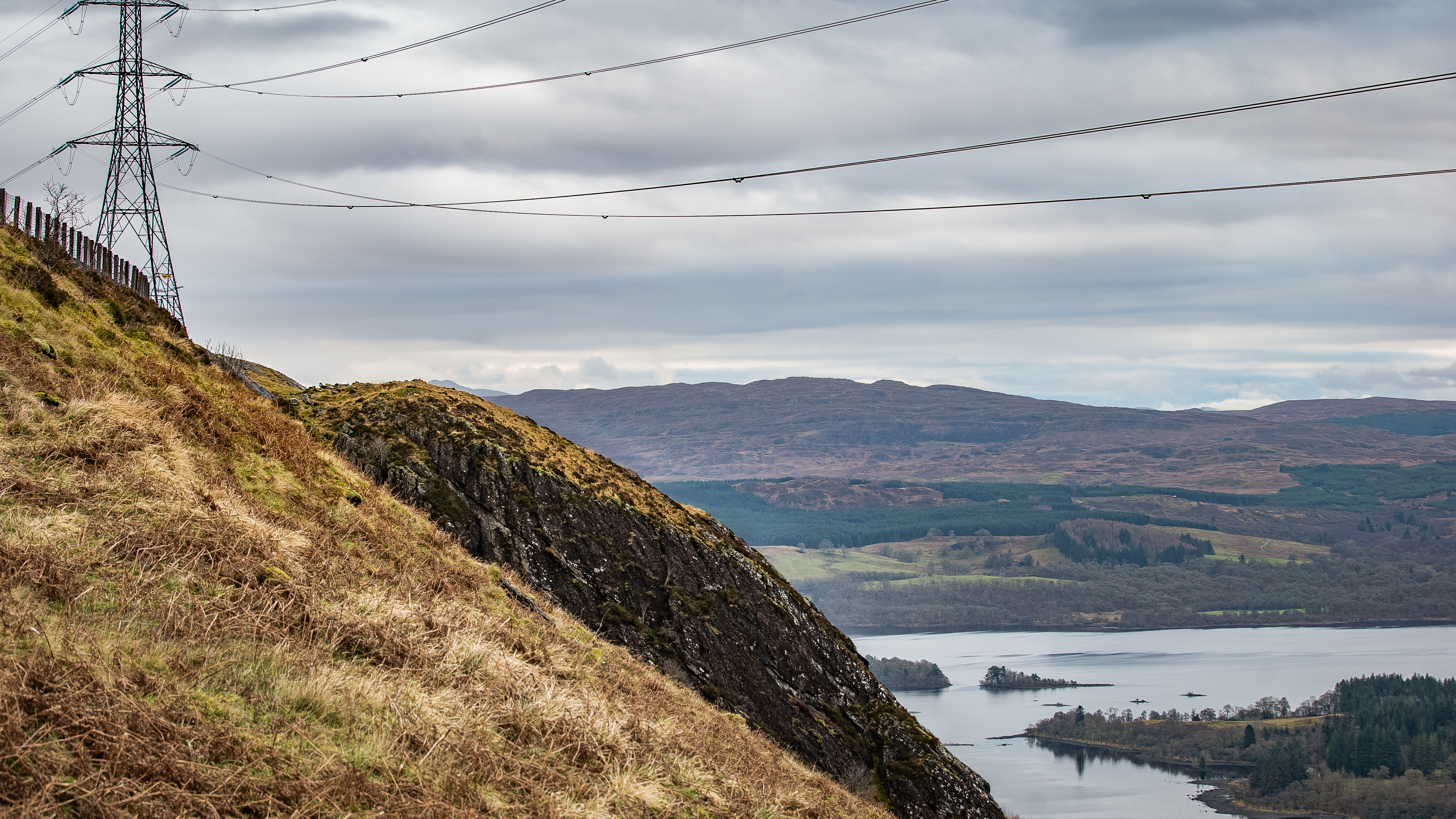
Electricity generation is often tied to a country’s geography, climate and geology. As an island Great Britain’s long coastline makes off-shore wind a key part of its renewable electricity, while Iceland can rely on its geothermal activity as a source of power and heat.
One of the most geographically-influenced sources of electricity is hydropower. A site needs a great enough volume of water flowing through it and the right kind of terrain to construct a dam to harness it. Even more dependent on the landscape is pumped hydro storage.
Pumped storage works by pumping water from one source up a mountain to a higher reservoir and storing it. When the water is released it rushes down the same shafts it was pumped up, spinning a turbine to generate electricity. The advantage of this is being able to store the potential energy of the water and rapidly deliver electricity to plug any gaps in generation, for example when the wind suddenly dropsor when Great Britain instantly requires a lot more power.
This specific type of electricity generation can only function in a specific type of landscape and the Scottish Highlands offers a location that ticks all the boxes.
The perfect spot for pumped storage
Cruachan Power Station, a pumped hydro facility capable of providing 440 megawatts (MW) of electricity, sits on the banks of Loch Awe in the Highlands, ready to deliver power in just 30 seconds.
“Here there is a minimum distance between the two water sources with a maximum drop,” says Gordon Pirie, Civil Engineer at Cruachan Power Station, “It is an ideal site for pumped storage.”
The challenge in constructing pumped storage is finding a location where two bodies of water are in close proximity but at severely different altitudes.
From the Lochside, the landscape rises at a dramatic angle, to reach 1,126 metres (3,694 feet) above sea level at the summit of Ben Cruachan, the highest peak in the Argyll. The crest of Cruachan Dam sits 400.8 metres (1,315 feet) up the slopes, creating a reservoir in a rocky corrie between ridges. The four 100+ MW turbines, which also act as pumps, lie a kilometre inside the mountain’s rock.
“The horizontal distance and the vertical distance between water sources is what’s called the pipe-to-length ratio,” explains Pirie. “It’s what determines whether or not the site is economically viable for pumped storage.”
The higher water is stored, the more potential energy it holds that can be converted into electricity. However, if the distance between the water sources is too great the amount of electricity consumed pumping water up the mountain becomes too great and too expensive.
The distance between the reservoir and the turbines is also reduced by Cruachan Power Station’s defining feature: the turbine hall cavern one kilometre inside the mountain…
Carving a power station out of rock
The access tunnel, cavern and the networks of passageways and chambers that make up the power station were all blasted and drilled by a workforce of 1,300 men in the late 1950s to early 1960s, affectionately known as the Tunnel Tigers.
This was dangerous work, however the rock type of the mountainside was another geographic advantage of the region. “It’s the diorite and phyllite rock, essentially granite, so it’s a hard rock, but it’s actually a softer type of granite, and that’s also why Cruachan was chosen as the location,” says Pirie.
The right landscape and geology was essential for establishing a pumped storage station at Cruachan, however, the West Highlands also offer another essential factor for hydropower: an abundance of water.
Turning water to power
The West Highlands are one of the wettest parts of Europe, with some areas seeing average annual rain fall of 3,500 millimetres (compared to 500 millimetres in some of the driest parts of the UK). This abundance of water from rainfall, as well as lochs and rivers also contributes to making Cruachan so well-suited to pumped storage.
The Cruachan reservoir can contain more than 10 million cubic metres of water. Most of this is pumped up from Loch Awe, which at 38.5 square kilometres is the third largest fresh-water loch in Scotland. Loch Awe is so big that if Cruachan reservoir was fully released into the loch it would only increase the water level by 20 centimetres.
However, the reservoir also makes use of the aqueduct system made up of 19 kilometres of tunnels and pipes that covers 23 square kilometres of the surrounding landscape, diverting rainwater and streams into the reservoir. Calculating quite how much of the reservoir’s water comes from the surrounding area is difficult but estimates put it at around a quarter.
“There are 75 concrete intakes dotted around the hills to gather water and carry it through the aqueducts to the reservoir,” says Pirie. “The smallest intake is about the size of a street drain in the corner of a field and the largest one is about the size of a three-bedroom house.”
Pumped storage stations offer the electricity system a source of extra power quickly but it takes the right combination of geographical features to make it work. Ben Cruachan just so happens to be one of the spots where the landscape makes it possible.
The different ways water powers the world
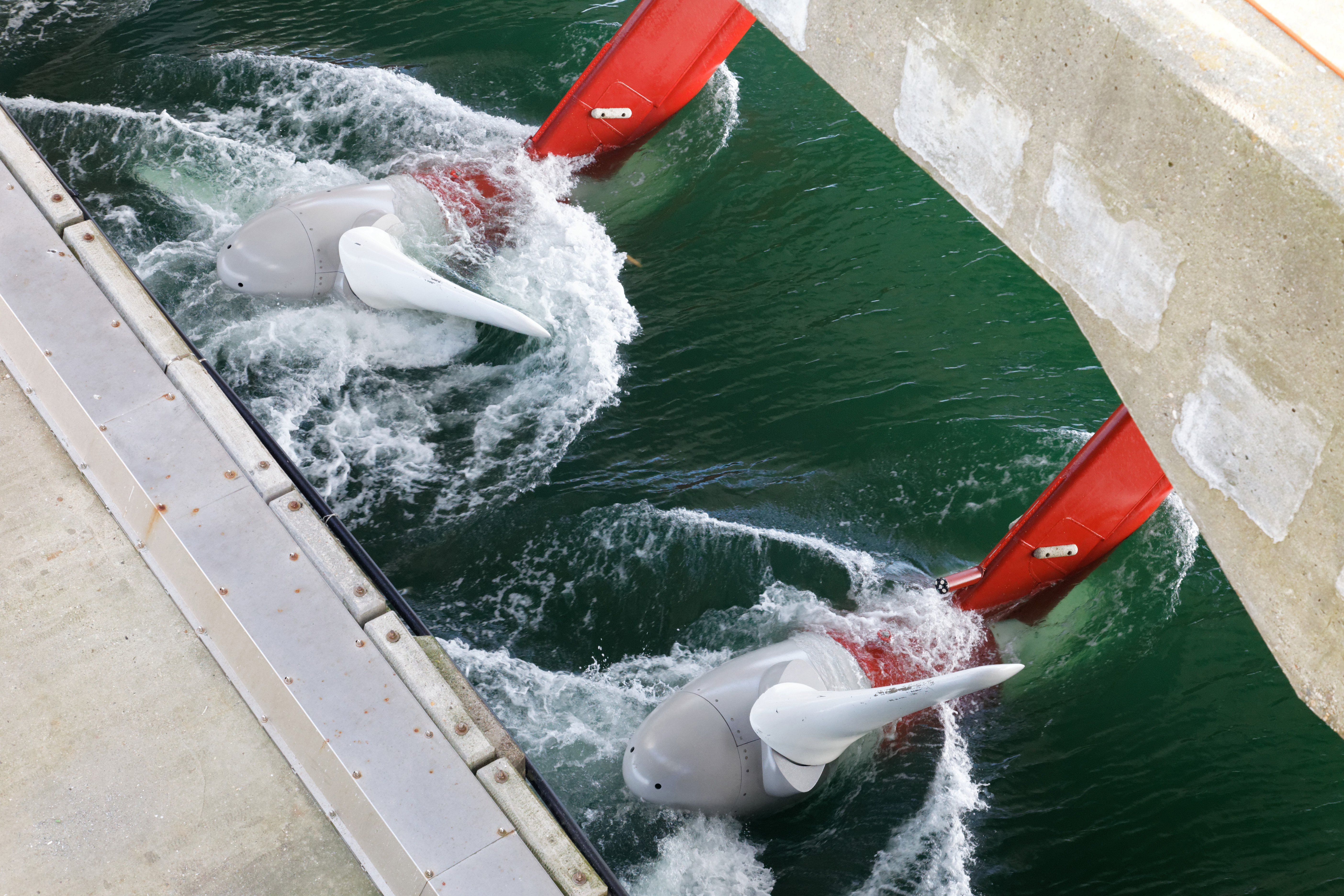
If the spectacular Roman aqueducts that still dot the landscape of Europe tell us anything, it’s that hydraulic engineering is nothing new. For thousands of years water power has been used to grind wheat, saw wood, and even tell the time.
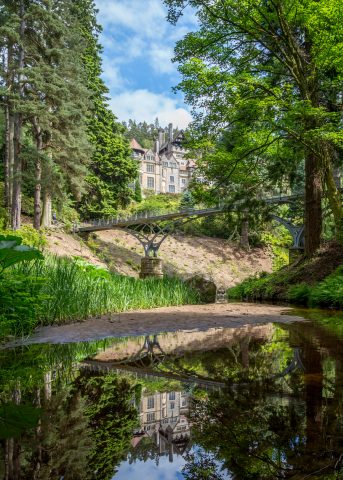
Craigside in Northumberland
By the 19th century, water was able to go beyond performing rudimentary mechanical tasks and generate electricity directly. Cragside in Northumberland, England became the first house powered entirely by hydroelectricity in 1878. By 1881, the whole town of Niagara Falls on the US-Canada border was being powered by the force of its eponymous river and waterfall.
Hydropower has many advantages: it’s predictable, consistent, often zero or low carbon and it can provide a range of ancillary services to power transmission systems. In Great Britain, there is 1.7 gigawatts (GW) of installed hydropower and another 2.8 GW of pumped hydro storage capacity, but it remains a small part of the overall electricity mix. In the fourth quarter of 2018, the 65% of British hydropower that is connected to the national grid accounted for less than one per cent electricity generation or 545,600 megawatt hours (MWh). By contrast, wind accounted for 14% of total generation that quarter (almost 9.5 million MWh).
While hydropower projects can be expensive to construct, operational and maintenance costs are relatively low and they can run for an extremely long time – the Lanark Hydro Electric Scheme in Scotland, which Drax recently acquired, has been producing power since 1927.
Today, hydropower installations are found at all scales, all around the world. But the term hydropower covers many different types of facility. These are some of the ways water is used to generate electricity.
Impoundment power plants
The simplest and most recognisable form of hydropower, impoundment facilities, work by creating a reservoir of trapped water behind a dam that is then selectively released, the water flows through a turbine, spinning it, which in turn activates a generator to produce electricity.
From the Hoover Dam on the Nevada-Arizona border, to the Three Gorges Dam in China – the world’s largest power station of any type, with a generating capacity of 22.5 GW – impoundment dams are some of the most iconic structures in modern engineering.
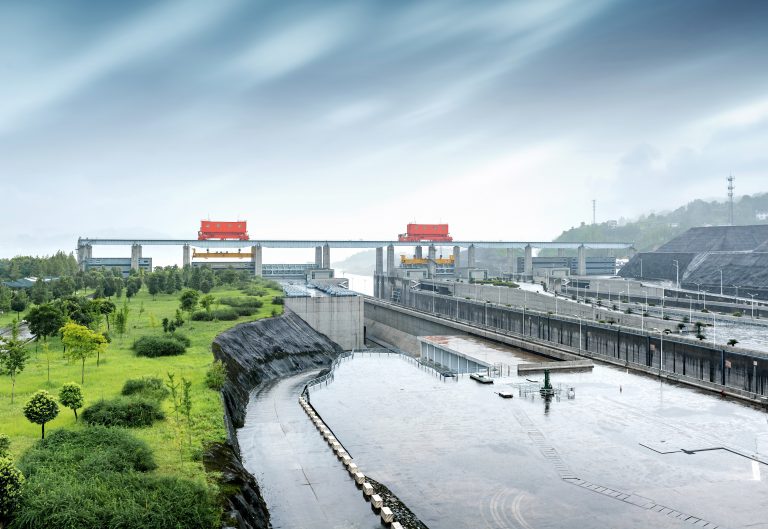
Three Gorges Dam, China
As well as having the potential to provide large quantities of baseload power, they can react extremely quickly to grid demands – just by opening or closing their floodgates as the power system operator requires.
Run-of-river generation
Rather than storing and releasing power from behind a dam, run-of-the-river generators channel off part of a river and use its natural flow to generate power.
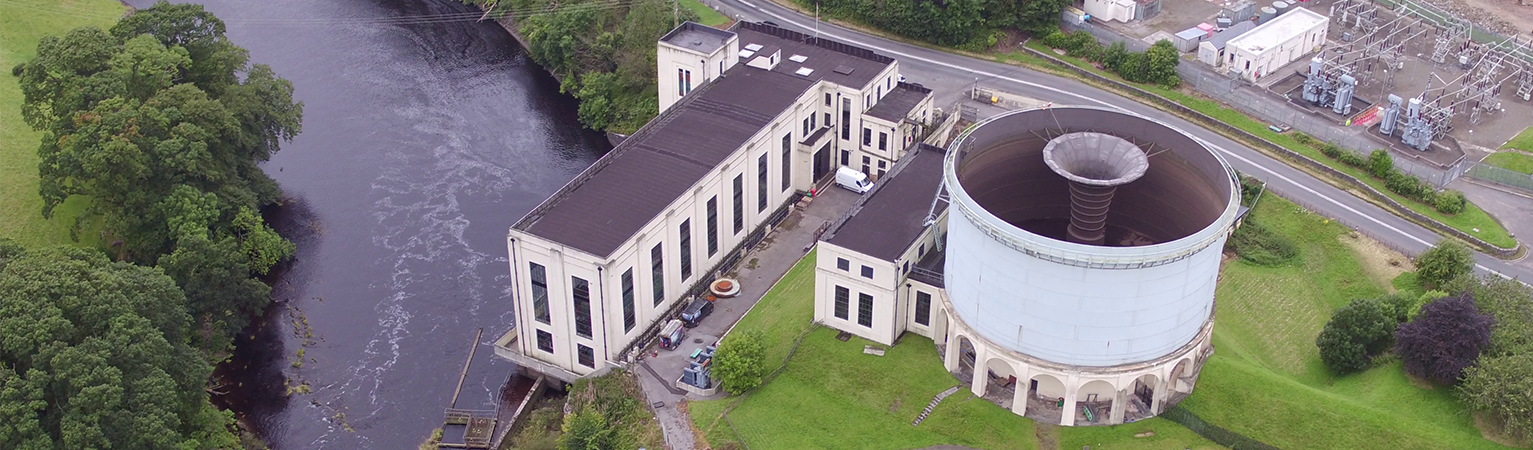
Tongland Power Station, Galloway Hydro Scheme
Because it doesn’t require large dams or reservoirs, run-of-river can be less environmentally disruptive, as there is not always a need for large scale construction and flooding is less common.
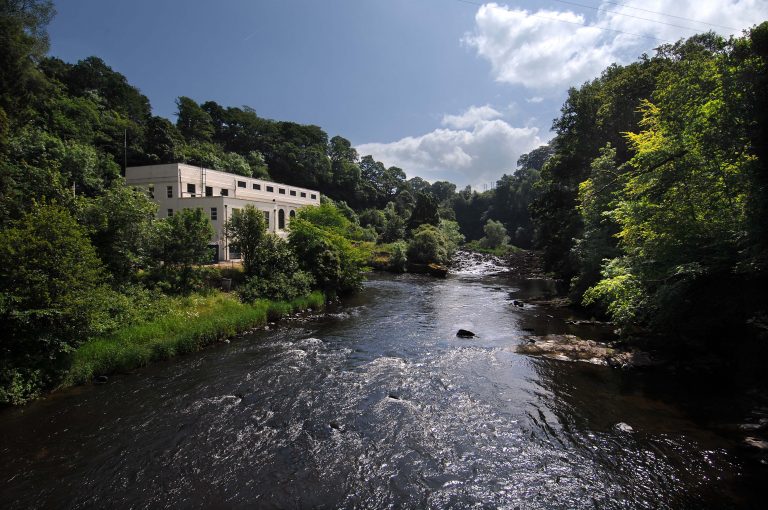
Stonebyres Power Station, Lanark Hydro Scheme
While run-of-river facilities tend to be smaller and less flexible than impoundment, they still have significant generating potential – the Jirau hydro-electric power plant on the Madeira river in Brazil has a generating capacity of 3.7 GW.
Pumped storage
Water can also be good for storing energy that can then be converted to electricity. Pumped hydro storage facilities operate by pumping water uphill to a reservoir when electricity is cheap or plentiful, then letting it flow back downhill through tunnels to a series of turbines that activate generators to generate electricity (in the same way as an impoundment dam) when electricity is in high demand.
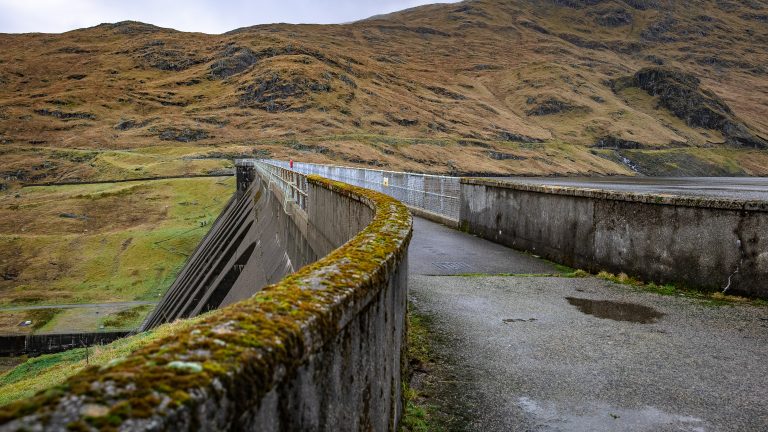
Dam and reservoir, Cruachan Power Station
Their ability to both produce and absorb electricity makes them a vital part of electricity networks, playing the role of energy storage systems. In fact, a massive 97% of all global grid storage capacity is in the form of pumped hydro. Their function as giant batteries will only become more important as intermittent renewable sources like wind and solar become more prevalent in the energy mix.
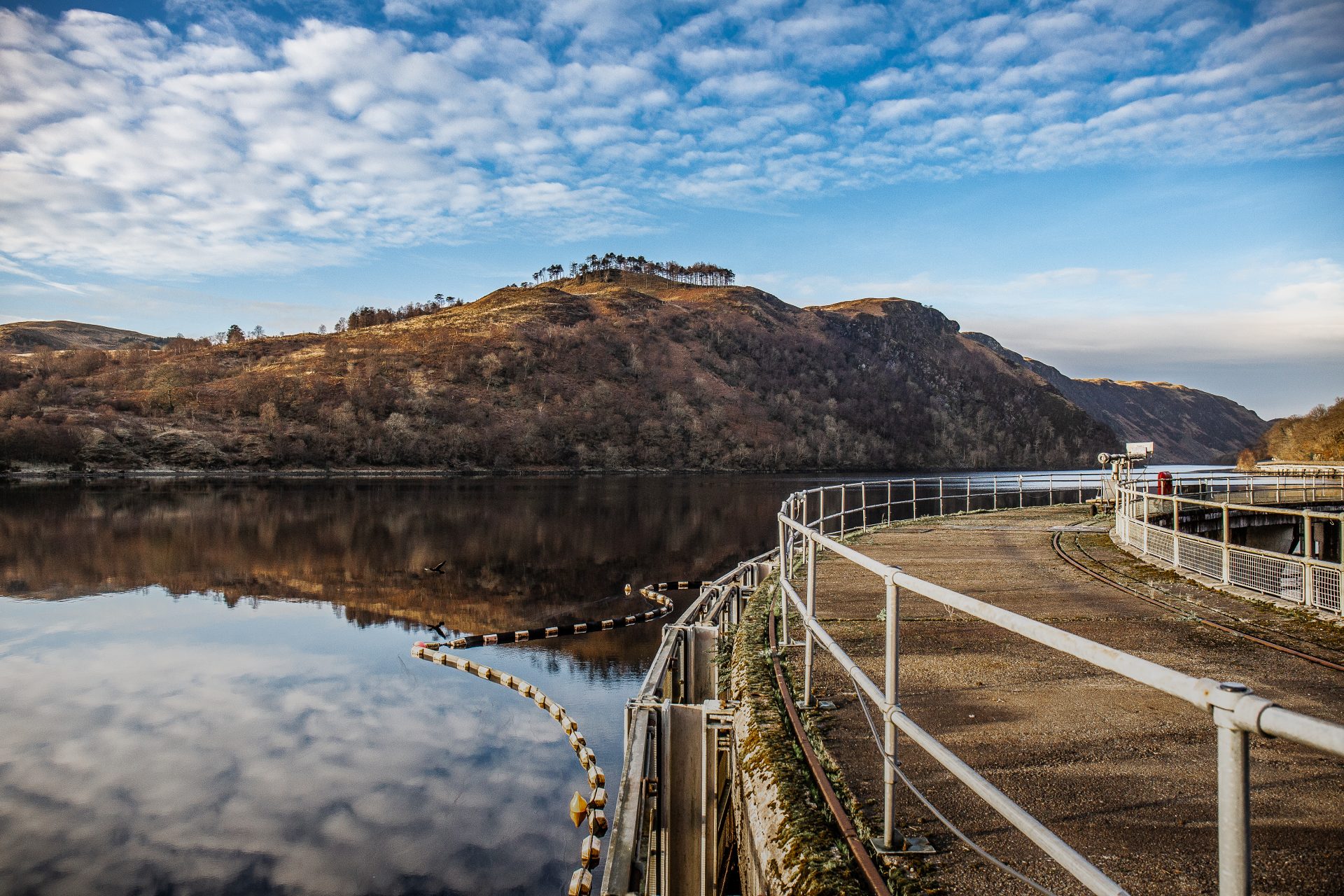
Outlet and loch, Cruachan Power Station.
So too will their ability to ramp up generation very quickly. Drax’s recently acquired Cruachan Power Station in Scotland can go from zero to 100 MW or more in less than 30 seconds when generation is called upon – for example, when there is a sudden spike in demand.
Tidal range generation
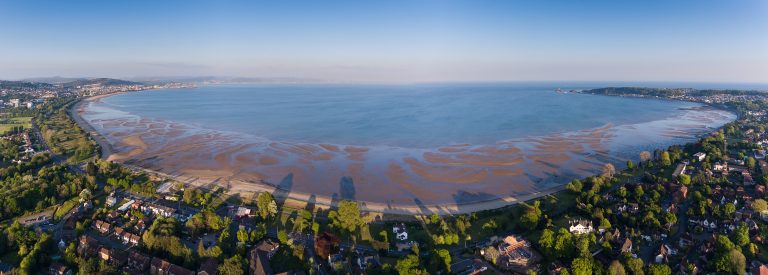
Swansea Bay
The sea is also an enormous source of potential hydropower. Tidal range generation facilities exploit the movement of water levels between and high and low tide to generate electricity. Tidal dams trap water in bays or estuaries at high tide, creating lagoons. The dam then releases the water as the rest of the tide lowers, allowing it to pass through turbines, generating power.
There are limitations – like wind and solar’s dependence on the wind blowing and the level of sunlight, operators can’t control when tides go in or out. But its vast generating potential means that it could be a valuable source of baseload power if it were to be deployed more widely.
Great Britain in particular has major opportunities for tidal generation. The Severn Estuary between England and Wales has the second highest tidal range in the world (15 metres), and a barrage built across the estuary could have a generating capacity of up to 8.6 GW – enough to meet 6% of the Britain’s total electricity demands. Some environmental groups worry about the impact such projects could have on wildlife.
Due to the level of public funding required, the government rejected that plan in 2010, in favour of pursuing its nuclear policy. A second attempt at securing a government-backed investment contract, known as a CfD, for a smaller 320 MW ‘pathfinder’ project in Swansea Bay was also rejected, in 2018. The Welsh government is however supportive of the project, which already has planning permission.
Tidal stream generation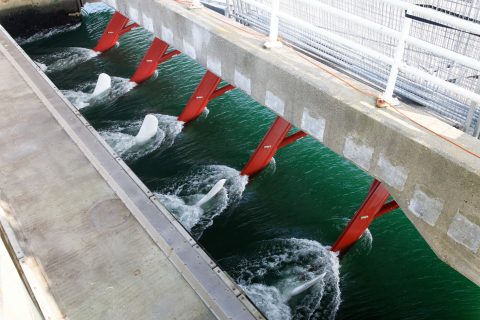
Rather than building a dam, tidal stream generators work like underwater wind turbines. Sturdy propellers or hydrofoils (wing-like blades which oscillate up and down rather than spinning around) are positioned underwater to transform the energy of tidal streams into electricity.
While tidal streams move far slower than wind, the high density of water compared to air means that more power is generated, even at much lower velocities.
Not reliant on large physical structures, tidal stream generators are a relatively cheap form of hydropower to deploy, and make a much smaller impact on their environment than tidal barrages.
Wave generation
Unlike tidal power, which is generated by the gravitational effects of the sun and moon on the Earth’s oceans, wave power ultimately comes from the winds that whip up the ocean’s surface.
There are a number of different methods that turn waves into generation, including funnelling waves into a tube floating on the surface of the water that contains electricity-generating turbines, or by using the vertical bobbing movement of a tethered buoy to pull and spin a fixed generator.
Wave power has yet to be widely implemented, but it has significant potential. It’s estimated that the waves off the coasts of the USA could have provided 66% of the country’s electricity generation needs in 2017 alone. Effectively commercialising wave power could provide another vital tool in developing a sustainable energy landscape for the coming future.
Tidal and wave power generation are less established generation technologies than their land-based cousins but they hold huge potential in delivering more sources of reliable, zero emissions electricity for energy systems in coastal locations around the world.
Can Great Britain keep breaking renewable records?
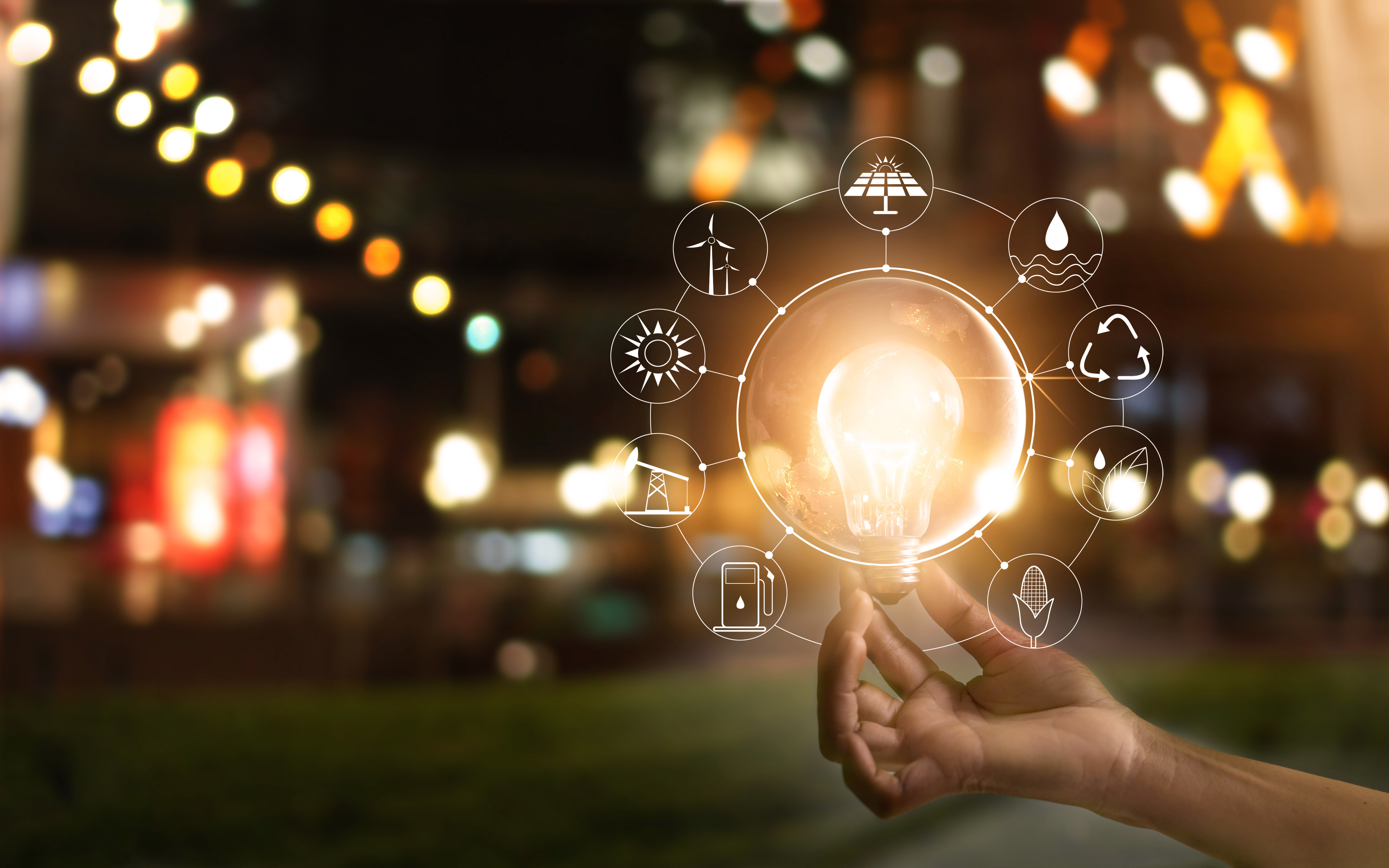
How low carbon can Britain’s electricity go? As low as zero carbon still seems a long way off but every year records continue to be broken for all types of renewable electricity. 2018 was no different.
Over the full 12-month period, 53% of all Britain’s electricity was produced from low carbon sources, which includes both renewable and nuclear generation, up from 50% in 2017. The increase in low carbon shoved fossil fuel generation down to just 47% of the country’s overall mix.
The findings come from Electric Insights, a quarterly report commissioned by Drax and written by researchers from Imperial College London.
The report found electricity’s average carbon intensity fell 8% to 217 grams of carbon dioxide per kilowatt-hour of electricity generated (g/kWh), and while this continues an ongoing decline that keeps the country on track to meet the Committee on Climate Change’s target of 100 g/kWh by 2030, it was, however, the slowest rate of decline since 2013.
It also highlights that while Britain can continue to decarbonise in 2019, the challenges of the years ahead will make it tougher to continue to break the records it has over the past few years.
The highs and lows of 2018
Last year, every type of renewable record that could be broken, was broken. Wind, solar and biomass all set new 10-year highs for respective annual, monthly and daily generation, as well as records for instantaneous output (generation over a half-hour period) and share of the electricity mix. The result was a new instantaneous generation high of 21 gigawatts (GW) for renewables, 58% of total output.
Wind had a particularly good year of renewable record-setting. It broke the 15 GW barrier for instantaneous output for the first time and accounted for 48% of total generation during a half hour period at 5am on 18 December.
Overall low carbon generation, which takes into account renewables and nuclear (both that generated in Britain and imported from French reactors), had an equally record-breaking year with an average of almost 18 GW across the full year and a new record for instantaneous output of 30 GW at 1pm on 14 June – nearly 90% of total generation over the half hour period.
While low carbon and individual renewable electricity sources hit record highs, there were also some milestone lows. Coal accounted for an average of just 5% of electricity output over the year, hitting a record low in June, when it made up just 1% of that month’s total generation. Fossil fuel output overall had a similarly significant decline, hitting a decade-low of 15 GW on average for 2018 – 44% of total generation over the year.
One fossil fuel that bucked the trend, however, was gas, which hit an all-time output of 27 GW for instantaneous generation on the night of 26 January. There was low wind on that day last year, plus much of the nuclear fleet was out of action for reactor maintenance. In one case, with seaweed clogging a cooling system.
This was all aided by an ongoing decline in overall demand as ever smarter and more efficient devices helped the country reach the decade’s lowest annual average demand of 33.5 GW. More impressive when considering how much the country’s electricity system has changed over the last decade, however, is the record low demand net of wind and solar. Only 9.9 GW was needed from other energy technologies at 4am on 14 June.
How the generation mix has changed
The most remarkable change in Britain’s electricity mix has been how far out of favour coal has fallen. From its position as the primary source from 2012 to 2014, in the space of four years it has crashed down to sixth in the mix with nuclear, wind, imports, biomass and gas all playing bigger roles in the system.
This sudden decline in 2015 was the result of the carbon price nearly doubling from £9.54 to £18.08 per tonne of carbon dioxide (CO2) in April, making profitable coal power stations loss-making overnight. With coal continuing to crash out of the mix, biomass has become the most-used solid fuel in Britain’s electricity system.
Interconnectors are also playing a more significant part in Britain’s electricity mix since their introduction to the capacity market in 2015. Thanks to increased interconnection to Europe, Britain is now a net importer of electricity, with 22 TWh brought in from Europe in 2018 – nine times more than it exported.
While more of Britain’s electricity comes from underwater power lines, less of it is being generated by water itself. Hydro’s decline from the fifth largest source of electricity to the eighth is the most noticeable shift outside coal’s slide. New large-scale hydro installations are expensive and a secondary focus for the government compared with cheaper renewables.
Hydro’s role in the electricity mix is also affected by drier, hotter summers, which means lower water levels. For solar, by contrast, the warmer weather will see it play a bigger role and it’s expected to overtake coal in either 2019 or 2020.
What is unlikely to change in the near-future, however, is the position at the top. In 2018 gas generated 115 TWh – more than nuclear and wind combined. But this is just one constant in a future of multiple moving and uncertain parts.
2019: a year of unpredictability
Britain is on course to leave the EU on 29 March. The effects this will have on the electricity system are still unknown, but one influential factor could be Britain’s exit from the Emissions Trading Scheme (ETS), the EU-wide market which sets prices of carbon emitted by generators. This may mean that rather than paying a carbon price on top of the ETS, as is currently the case, Britain’s generators will only have to pay the new, fixed carbon tax of £16 per tonne the UK government says will come into play in April, topped up by the carbon price support (CPS) of £18/tonne.
Lower prices for carbon relative to the fluctuating ETS + CPS, could make coal suddenly economically viable again. The black stuff could potentially become cheaper than other power sources. This about-turn could cause the carbon intensity of electricity generation to bounce up again in one or more years between 2019 and 2025, the date all coal power units will have been decommissioned.
The knock-on effect of lower carbon prices, combined with fluctuations in the Pound against the Euro, could see a reverse from imports to exports as Britain pumps its cheap, potentially coal-generated, electricity over to its European neighbours. That’s if the interconnectors can continue to function as efficiently as they do at present, which some parties believe won’t be the case if human traders have to replace the automatic trading systems currently in place.

Sizewell B Nuclear Power Station
A reversal of importing to exporting could also reduce the amount of nuclear electricity coming into the country from France. Future nuclear generation in Britain also looks in doubt with Toshiba and Hitachi’s decisions to shelve their respective plans for new nuclear reactors, which could leave a 9 GW hole in the low-carbon base capacity that nuclear normally provides.
Renewables have the potential to fill the gap and become an even bigger part of the electricity system, but this will require a push for new installations. 2018 saw a 60% drop in new wind and solar installations and less than 2 GW of new renewable capacity came onto the system, making it the slowest year for renewable growth since 2010.
Britain’s electricity has seen significant change over the last decade and 2018 once again saw the country take significant strides towards a low carbon future, but challenges lie ahead. Records might be harder to break, but it is important the momentum continues to move towards renewable, sustainable electricity.
Explore the quarter’s data in detail by visiting ElectricInsights.co.uk. Read the full report.
Commissioned by Drax, Electric Insights is produced, independently, by a team of academics from Imperial College London, led by Dr Iain Staffell and facilitated by the College’s consultancy company – Imperial Consultants.
Electricity and magnetism: the relationship that makes the modern world work
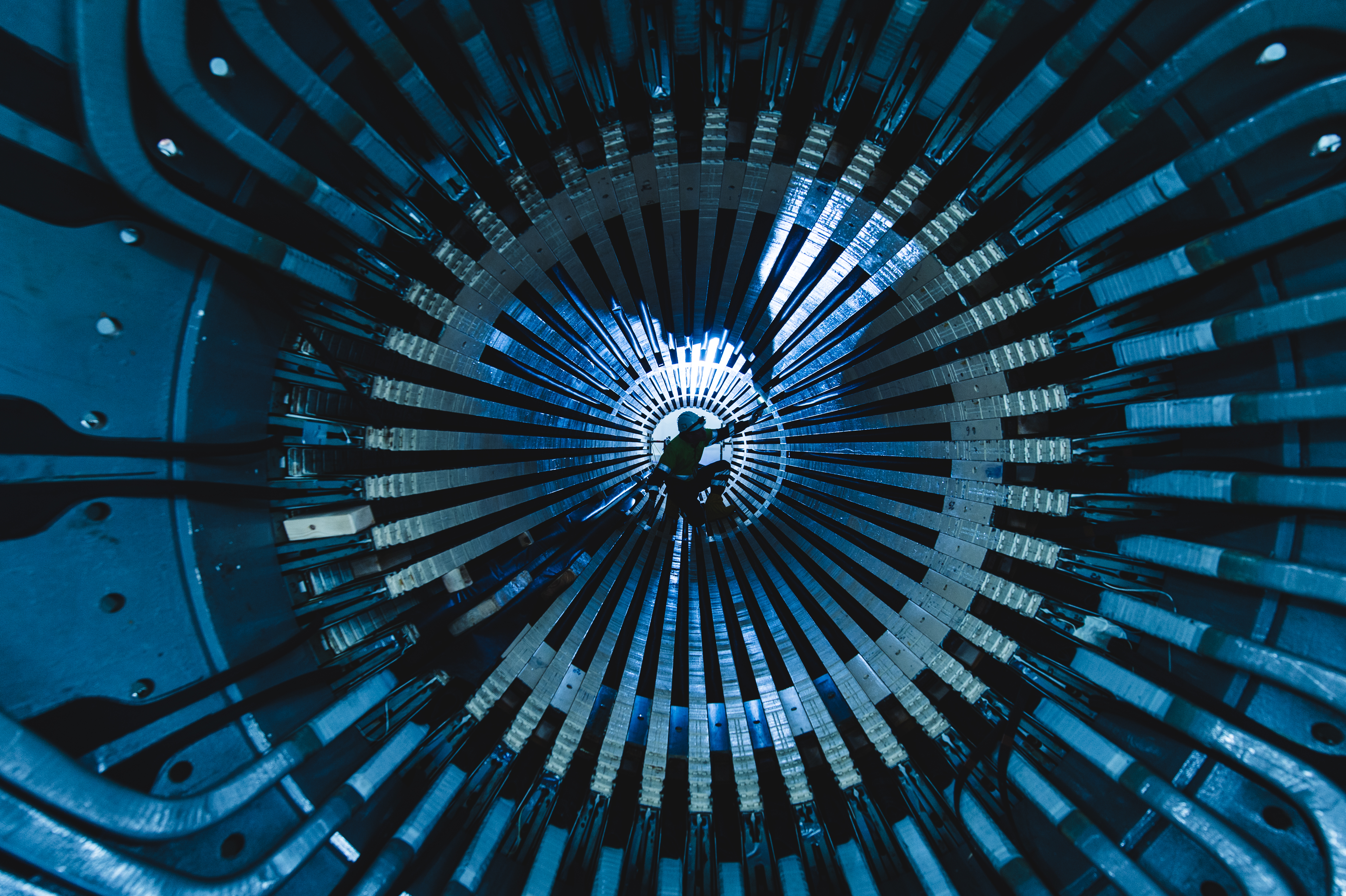
Locked in a Parisian vault and stored in a double set of bell jars is a small cylinder of metal. Made of platinum-iridium, the carefully guarded lump weighs exactly one kilogram. But more than just weighing one kilogram, it is the kilogram from which all other official kilograms are weighed.
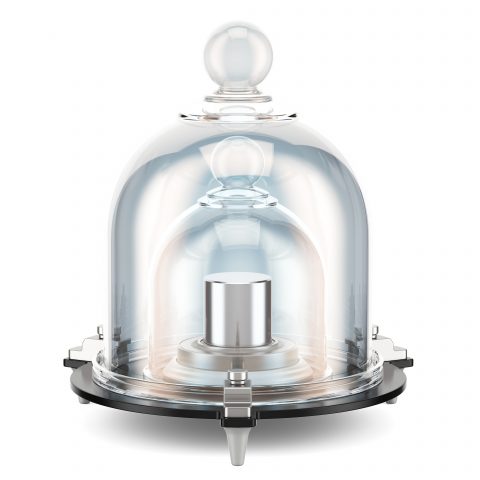
International prototype kilogram with protective double glass bell
Known as the International Prototype Kilogram, or colloquially as Le Grand K, the weight was created in 1889 and has been carefully replicated to offer nations around the world a standardised kilogram. But over time Le Grand K and its clones have slightly deteriorated through wear and tear, despite extremely careful use. In an age of micro and nanotechnology, bits of metal aren’t quite accurate enough to dictate global weighs and so as of May this year it will no longer be the global measurement for a kilogram. An electromagnet is part of its replacement.
An electromagnet is effectively a magnet that is ‘turned on’ by running an electric current through it. Cutting the current turns it off, while increasing or decreasing the strength of the current increases and decreases the power of the magnet.
It can be used to measure a kilogram very precisely thanks to something called a Kibble Balance, which is essentially a set of scales. However, instead of using weights it uses an electromagnet to pull down one side. Because the electric current flowing through the electromagnet can be increased, decreased and measured very, very accurately, it means scientists can define any weight – in this case a kilogram – by the amount of electrical current needed to balance the scale.
This radical overhaul of how weights are defined means scientists won’t have to fly off to Paris every time they need precise kilograms. Beyond just replacing worn-out weights, however, it highlights the versatility and potential of electromagnets, from their use in electricity generation to creating hard drives and powering speakers.
The simple way to make a magnet
Magnets and electricity might at first not seem closely connected. One powers your fridge, the other attaches holiday souvenirs to it. The former certainly feels more useful. However, the relationship between magnetic and electric fields is as close as two sides of the same coin. They are both aspects of the same force: electromagnetism.
Electromagnetism is very complicated and there’re still aspects of it that are unknown today. It was thinking about electromagnetism that led Einstein to come up with his theory of special relativity. However, actually creating an electromagnet is relatively straightforward.
All matter is made up of atoms. Every neutral atom’s core is made up of static neutrons and protons, with electrons spinning around them. These electrons have a charge and a mass, giving the electrons a tiny magnetic field. In most matter all atoms are aligned in random ways and effectively all cancel each other out to render the matter non-magnetic. But if the atoms and their electrons can all be aligned in the same direction then the object becomes magnetic.
A magnet can stick to an object like a paperclip because its permanent magnetic field realigns the atoms in the paperclip to make it temporarily magnetic too – allowing the magnetic forces to line up and the materials to attract. However, once the paper clip is taken away from the magnet its atoms fall out of sync and point in random directions, cancelling out each other’s magnetic fields once again.
Whether a material can become magnetic or not relies on a similar principal as to whether it can conduct electricity. Materials like wood and glass are poor conductors because their atoms have a strong hold over their electrons. By contrast, materials like metals have a loose hold on their electrons and so are good conductors and easily magnetised. Nickle, cobalt and iron are described as ferromagnetic, because their atoms can stay in sync making them a permanent magnet. But when magnets really become useful is when electricity gets involved.
Putting magnets to work
Running an electric current through a material with a weak hold on its electrons causes them to align, creating an electromagnetic field. Because of the relationships between electric and magnetic fields, the strength of the electromagnet can also be altered by increasing or decreasing the current, while switching the flow of the current will flip its north and south poles.
Having this much control over a magnetic field makes it very useful in everyday life, including how we generate electricity.
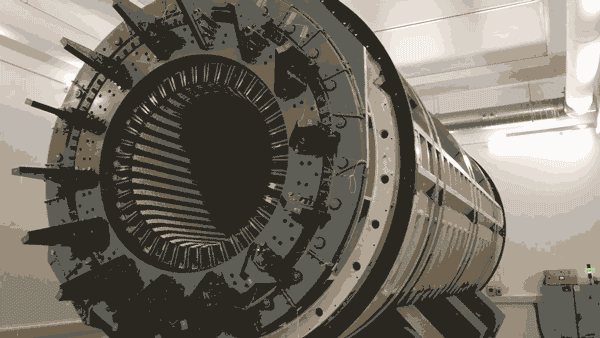
Find out how we rewind a generator core in a clean room at the heart of Drax Power Station
Inside each of the six generator cores at Drax Power Station, is a 120-tonne rotor. When a voltage is applied, this piece of equipment becomes a massive electromagnet. When steam powers the turbines to rotate it at 3,000 rpm the rotor’s very powerful magnetic field knocks electrons in the copper bars of the surrounding stator out of place, sending them zooming through the metal, in turn generating an electrical current that is sent out to the grid. The 660 megawatts (MW) of active power Drax’s Unit 1 can export into the national transmission system is enough to power 1.3 million homes for an hour.
Beyond just producing electricity, however, electromagnets are also used to make it useful to everyday life. Almost anything electric that depends on moving parts, from pumping loud speakers to circuit breakers to the motors of electric cars, depend on electromagnets. As more decarbonisation efforts lead to greater electrification of areas like transport, electromagnets will remain vital to daily life into the future.
How to get more EVs on the roads
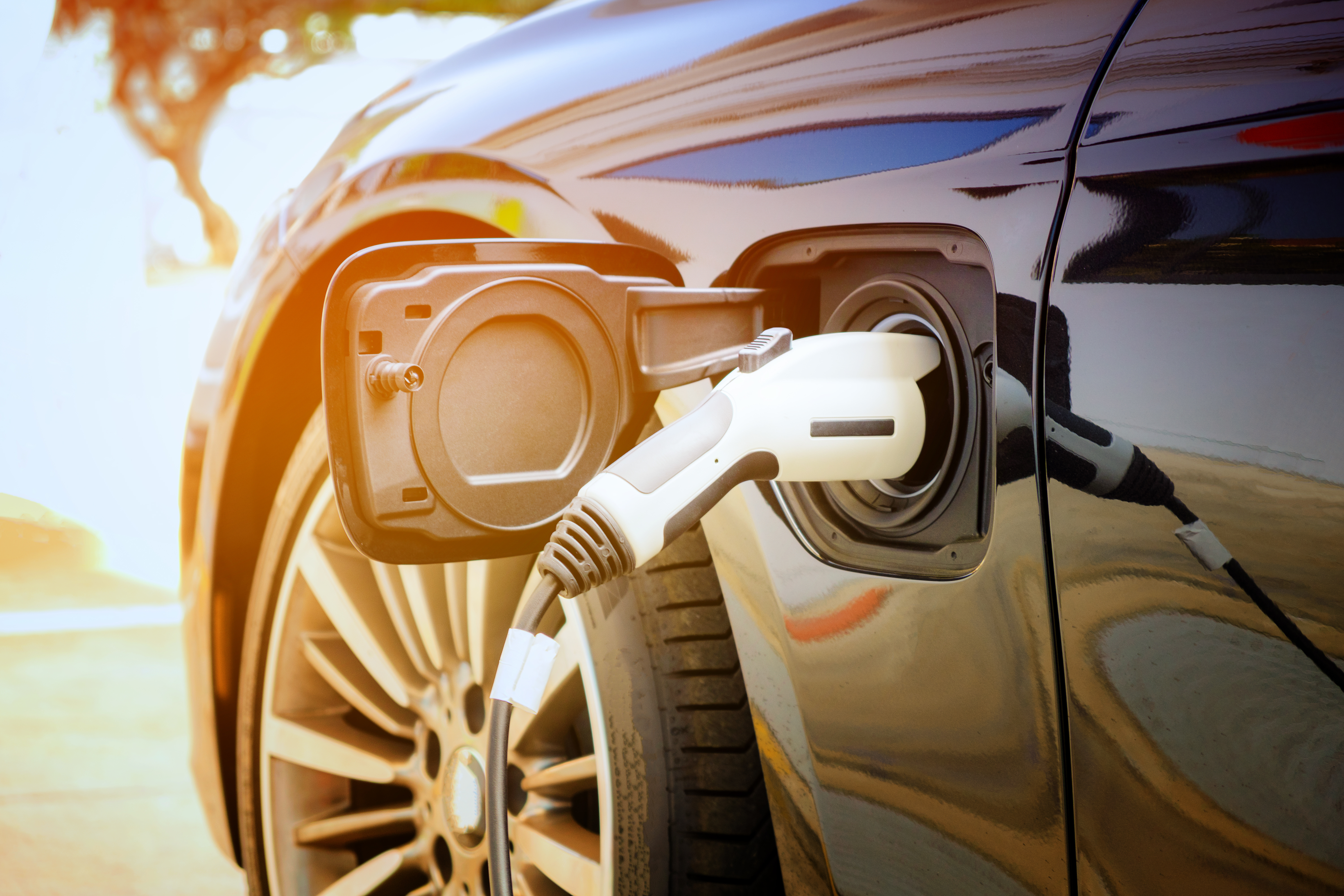
From school runs to goods deliveries, getting from A to B is crucial to life in modern Britain. However, a progress report by the Committee on Climate Change (CCC) found that in 2017 transport was the largest greenhouse gas (GHG)-emitting sector in the UK, accounting for 28% of total emissions. Within domestic transport, cars, vans and HGVs are the three most significant sources of emissions, accounting for 87% of the sector’s emissions.
A zero carbon future relies on a major shift away from petrol and diesel engines to electric transport. A recent report, Energy Revolution: A Global Outlook, by academics from Imperial College London and E4tech, commissioned by Drax, examines the decarbonisation efforts of 25 major countries. The report found the UK ranked sixth in sales of new electric vehicles (EVs) in the 12 months to September 2018 and seventh for the number of charging points available.
The government’s Road to Zero strategy outlines the country’s target for as many as 70% of new car sales to be ultra-low emission by 2030, alongside up to 40% of new vans. It has, however, been criticised by the Committee on Climate Change as not being ambitious enough. A committee of MPs has suggested 2032 becomes the official target date for banning new petrol and diesel cars, rather than 2040 called for in the strategy.
Even as the range of EVs on the market grows, getting more low-emission vehicles on roads will require incentives and infrastructure improvements. Here’s how some of the countries leading the shift to electrified transport are driving adoption.
Expanding charging infrastructure
One of major barriers to EV adoption is a lack of public charging facilitates, coupled with reliability issues across a network that includes both old hardware and a plethora of apps and different connections. No one wants to set off on a long journey unsure of whether they’ll be able to find a recharging point before their battery goes flat.
According to the Energy Revolution report, there is one charger for every 5,000 people in the UK, compared to one for every 500 people in Norway, the leading country for charging points. The Scandinavian country’s government has invested heavily in its policy of placing two fast charging stations for every 50 km of main road, covering 100% of the cost of installation.
Government support has also been crucial in second and third ranked countries, The Netherlands and Sweden, respectively. The Dutch Living Lab Smart Charging is a collaboration between government and private organisations to use wind and solar to change vehicles. While Sweden has combined its ‘Klimatklivet’ investment scheme for both public and private charge points, with experiments, such as charging roads.
China, where half of the world’s 300,000 charge points are located, has issued a directive calling for the construction of 4.8 million electric charging points around the country by 2020. It’s also assisting private investments to make charging stations more financially viable.
The UK’s Road to Zero Strategy is to expand charging infrastructure through a £400 million joint investment fund with private investors.
Drax’s Energising Britain report found the UK is on track to meet its 2030 target of 28,000 installed chargers ahead of time. However, deployment still clusters around London, the South East and Scotland.
More direct government incentives or policies may be needed to balance this disparity and in the UK, the Scottish Government is leading the pack with a 2032 ban on new petrol and diesel cars plus a range of initiatives including public charging networks and the Switched on Towns and City Fund.
Charging points are necessary for electrified roads. However, it’s a chicken-and-egg situation –more chargers don’t mean more EVs. Getting more EVs on roads also requires financial incentive.
Money makes the wheels go around
Putting infrastructure in place is one thing, but the reality is EVs are expensive, especially new ones and cold hard cash is an important driver of adoption.
Financial incentives have been a part of Norway’s policies since the 1980s, with the country’s high fuel prices, compared to the US for example, further helping to make EVs attractive. Current benefits for EV owners include: no import or purchase taxes, no VAT, no road tax, no road tolls, half price on ferries and free municipal parking. There are also non-financial incentives such as bus-lane usage.
Sweden, the second ranked country for new EV sales in 2018, is a similar case where high fuel prices are combined with a carrot-and-stick approach of subsidies for EVs and rising road taxes for fossil fuel-powered vehicles, including hybrids.
The UK has had a grant scheme in place since 2011, but last year removed hybrid vehicles from eligibility and dropped the maximum grant for new EV buyers from £4,500 to £3,500. EVs are also exempt from road taxes. In April 2019, Transport for London is implementing a Low Emissions Zone (ULEZ) which exempts EVs from a daily charge.
Subsidies for both buyers and vehicle manufacturers have been a cornerstone of China’s policies, with support coming up to around $15,000 per vehicle. Chinese EV buyers can also skip the lottery system for new license plates the country has in place to reduce congestion.
Heavy subsidies have allowed the country to claim as much as 50% of the entire EV passenger market, however, it makes change expensive and the government is now preparing to find a more sustainable way of driving adoption.
Preparing for transport beyond subsidies
China isn’t afraid to strong-arm manufacturers into building more EVs. Companies with annual sales of more than 30,000 vehicles are required to meet a quota of at least 10% EVs or hybrids. However, the government has begun to scale back subsidies in the hope it will drive innovation in areas such as batteries, robotics and automation, which will in turn reduce the price for end consumers.
Norway, which owes so much of its decarbonisation leadership in low-carbon transport to subsidies, is also grappling with how to move away from this model. As EVs creep increasingly towards the norm, the taxes lost through EV’s exclusions become more economically noticeable. While the government says the subsidies will remain in place until at least 2020, different political parties are calling to make the market commercially viable.
There is also concern the schemes only pass on savings to those who can afford new EV models, rather than the wider population, who face higher taxes for being unable to upgrade.
It’s not just governments’ responsibility to make new markets for EVs sustainable, but for business to innovate within the area too. Drax Group CEO Will Gardiner recently said his company must help to “ensure no-one is left behind through the energy revolution”.
That’s a view welcomed by politicians from all sides of the political spectrum concerned not just about mitigating man-made climate change but also to ensure a ‘just transition’ during the economy’s decarbonisation.
Energy and Clean Growth Minister Claire Perry spoke at an Aldersgate Group event in London in January:
“It’s been very easy, in the past, for concerns about the climate to be dismissed as the worries of the few, not the many. Luckily, we’ve been able to strip out a lot of the myths surrounding decarbonisation and costs –but we have to be mindful that this is a problem which will have to be solved by the many, not just the middle class.”
Many countries have set ambitious targets for when the ban of new petrol and diesel vehicles will come into effect. Government involvement and subsidies will be crucial but may prove economically challenging in the longer term.
Explore the full reports:
Energy Revolution: A Global Outlook
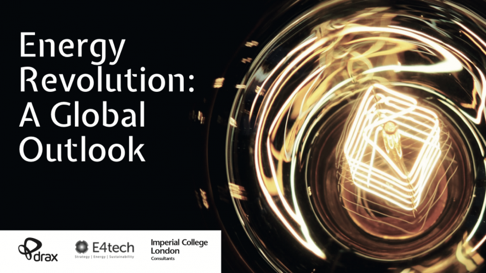
I. Staffell, M. Jansen, A. Chase, E. Cotton and C. Lewis (2018). Energy Revolution: Global Outlook. Drax: Selby.
Energising Britain: Progress, impacts and outlook for transforming Britain’s energy system
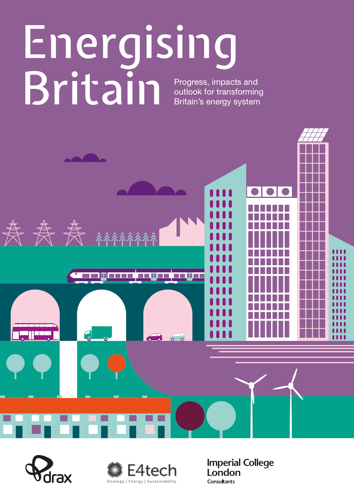
I. Staffell, M. Jansen, A. Chase, C. Lewis and E. Cotton, (2018). Energising Britain: Progress, impacts and outlook for transforming Britain’s energy system. Drax Group: Selby.